ABSTRACT:
Largemouth bass (LMB; ~15.2 g initial weight) was fed one of the five experimental, and a fishmeal (FM)/fish oil (FO) control diet and two commercial (Xinxin, Coppens) LMB feeds for 10 weeks. Fish were dispersed into 24, 110-Laquaria (n = 20/tank) configured as a recirculating system. Aquaria were arbitrarily allocated to dietary treatments in triplicate (n = 60/fish diet). The FM component of Experimental diets was replaced using poultry by-product meal and soy protein concentrate. One experimental dietcontained FO, whereas in others, FO was replaced with canola,flax and/or algal oil or combinations thereof. At trial end, there were no differences among fish-fed various diets in terms of feed conversion or protein efficiency ratios. Weight gain and survival were similar for experimental groups, and LMB fed the Xinxin commercial feed, but the Coppens feed returned inferior (p < 0.05) weights and higher mortality. Condition factor, fillet yield and intraperitoneal fat ratios were unaffected by diet but hepatosomatic index varied. The Coppens feed caused greatest differences in whole-bodyprotein, lipid and ash contents (p < 0.001). Whole-body fatty acid profiles reflected those of the diet, with algal oil enriched feed illustrating enhanced DHA:EPA ratios.
1 | INTRODUCTION
Global aquaculture production of Perciformes, or perch-like fishes, exceeds 818,000 tonnes, representing approximately 0.7% of total tonnage (FAO, 2020). As the 18th ranked farmed order, their worth, at around US$ 7 billion, however, places them 9th among all other groups, thereby underscoring their high value (Cai et al., 2019). The principal cultivated species, at over 50% of the total, is the largemouth bass (LMB), Micropterus salmoides. While farmed in a half dozen countries, it is China that accounts for the vast majority (Hussein et al., 2020), which, in 2018, stood at 432,000 tonnes (CFS, 2019). An important component of increased LMB production has been farmers switching from tilapia due, among other factors, to its higher market price. However, several issues presently hinder the future expansion of LMB production in China and elsewhere. For example, the emergence of various viral and bacterial diseases has taken their toll on production (Gui & Zhang, 2018; Lei et al., 2020; Xia et al., 2018). The lack of genetically improved strains, and poor seed quality, production and distribution, have likewise been recognized as obstructive to industry growth (Bai & Li, 2018; Hussein et al., 2020). Disproportionate regional production, the need for improved methods of cultivation, volatility in market prices and lack of branding are also regarded as problematic (Bai & Li, 2018; Wang et al., 2020).
Like other farmed carnivorous fishes, LMB has a high dietary protein requirement (Cai et al., 2020), which is generally provided in large part by fishmeal (FM). FM is favoured as the protein feedstuff of choice because of its high nutrient density, excellent profile of essential amino acids, high palatability and digestibility (National Research Council, 2011). As the mainstay of manufactured feeds for decades (Naylor et al., 2009), FM is also a source of nucleotides, phospholipids, essential fatty acids, vitamins and minerals. Nonetheless, FM quality can vary with season, origin, storage and processing (Sharma et al., 2019), and periodic fluctuations in availability due to climate-related events and overfishing already impact pricing (Pincinato et al., 2020). Moreover, it has been suggested that if current use of FM and fish oil (FO) by the animal feed sectors remain the same, forage fish populations will be overextended by 2050, or before (Froehlich et al., 2018; Konar et al., 2019). Such a scenario will undoubtedly result in even higher raw material costs. Already FM is expensive relative to other terrestrial sources of protein.For example, the price of FM has increased 3.4-fold over the last 20 years, and the current cost is around $1443–1720 per tonne, depending on origin and quality (Bacon, 2020; Index Mundi, 2020). On the other hand, the price for soybean meal (SBM), an oft used substitute for FM in aquafeeds, has risen 2.8-fold over the same timeframe and is almost a third less at a present-day cost of $387 per tonne (Index Mundi, 2020).
In efforts to safeguard the supply of seafood, including that employed in reduction to FM for aquafeeds, China has undertaken a planned approach by expanding its deep-water fishing fleet and global operational range (Crona et al., 2020; Lillebø et al., 2017). In addition, bilateral agreements, and purchase of foreign companies and their quotas have been employed (Anonymous, 2016; Crona et al., 2020). Even with stable supplies of FM/FO, however, their use in commercial extruded pellets (Fan et al., 2020; Huang et al., 2017), in the main areas of LMB production, viz. Hubei, Guangdong (mainly Shunde and Nanhai districts) and Jiangsu (mainly Wujiang and Nanjing districts) Provinces (Ding et al., 2013; Hussein et al., 2020), accounts for over 60% of operational costs (Fan et al., 2020). For future economic and environmental viability and growth, the LMB aquaculture sector must replace these expensive ingredients with practical alternatives. Developing additional supply streams of nutritionally equivalent ingredients will allow feed formulators the optionality to create economical formulations despite changing markets and fluctuating prices. The sustainability imperative is given authority by consumers who demand safer and more ethically and environmentally responsive food production systems (Yi, 2019), underscored by their growing consternation over the potential contamination of FM/FO by heavy metals and persistent organic pollutants (Fink-Gremmels, 2012; Li et al., 2019) and, more recently, microplastics (Castelvetro et al., 2020; FAO, 2018). Focussing on consumer demands has commenced in earnest with the examination and use of byproducts and alternative proteins and oils from diverse sources (Gasco et al., 2018; Gatlin et al., 2007; Glencross et al., 2020; Han et al., 2018; Mo et al., 2018). In particular, soy, insect meal, bacteria and yeasts, and algae show potential to replace FM/FO and reduce global forage fish demand (Cottrell et al., 2020).
It is noteworthy that some alternative proteins and oils express nutrient imbalances (e.g. essential amino and fatty acids) and antinutritional factors (e.g. enzyme inhibitors, lectins, saponins and phytic acid) that can result in negative effects on feed palatability and digestibility, growth, physiology and metabolism (Colombo, 2020; Turchini et al., 2009). This is especially so when only a single source of alternate dietary protein or oil is used. Accordingly, the objective of the present study was to evaluate the response of juvenile LMB to dietary replacement of FM and FO using protein blends and alternative single source and blends of vegetable oils. Along with examining growth, survival and proximate composition, the effect of varying the source of oil on whole-body fatty acid profiles was also evaluated.
2 | MATERIALS A ND METHODS
2.1 | Diets
Two commercial feeds (Huifu, Xinxin Tian’en Company, Zhejiang Province, PR China and Alltech Coppens, Leende, The Netherlands), specifically formulated for LMB, were employed as reference diets.
Six experimental diets (Table 1) were manufactured, using twin-screw cooling extrusion (Wenger TX 57, Wenger Manufacturing, Sabetha, KS, USA), at Zeigler Brothers Inc. (Gardners, PA, USA), as 1-mm sinking pellets. After drying and cooling, all diets were placed in plastic-lined paper bags and shipped to Texas A&M University and stored in a cool dry location. Due to the small size of the pellets relative to the size of the fish, each experimental diet was mixed with carboxymethyl cellulose at 2% by weight, then moistened with water and passed through a meat grinder attachment of a Hobart mixer (Hobart Inc., Troy, OH, USA) with a 2-mm die plate to form pellets. A second batch of diet was prepared using the same procedure but passed through a 3-mm die plate. Equal amounts of each batch of experimental diet were combined and analyzed for proximate composition according to established procedures (AOAC, 2005). The results of those analyses are summarized in Table 2. The fatty acid composition of lipids used to prepare the experimental diets and the analyzed diets are provided in Tables 3 and 4, respectively.
2.2 | Culture system and procedures
Procedures used in this study were approved by the Texas A&M University System Institutional Animal Care and Use Committee. Experimental diets were evaluated using 110-L aquaria configured in a recirculating aquaculture system (RAS) located within a climate-controlled laboratory as described previously (Rosales et al., 2017; Wu et al., 2015). Feed-trained LMB juveniles were obtained from a commercial facility (Dunn’s Fish Farms, Inc., Brinkley, AR, USA) and acclimated to the RAS during a 2-week conditioning period by feeding a commercial diet (Huifu, Xinxin Tian’en Company, Zhejiang Province, PR China). At the initiation of the feeding trial, fish were sorted into a similar size range (initially averaging 15.2 g each) with groups of 20 per aquarium stocked into each aquarium. Each diet was randomly assigned to three replicate aquaria, and the feeding trial continued for a total of 10 weeks. Fish in each aquarium were fed their respective diets twice daily by allowing them to actively
TABLE 1 Ingredient composition of experimental diets for largemouth bass (Micropterus salmoides)
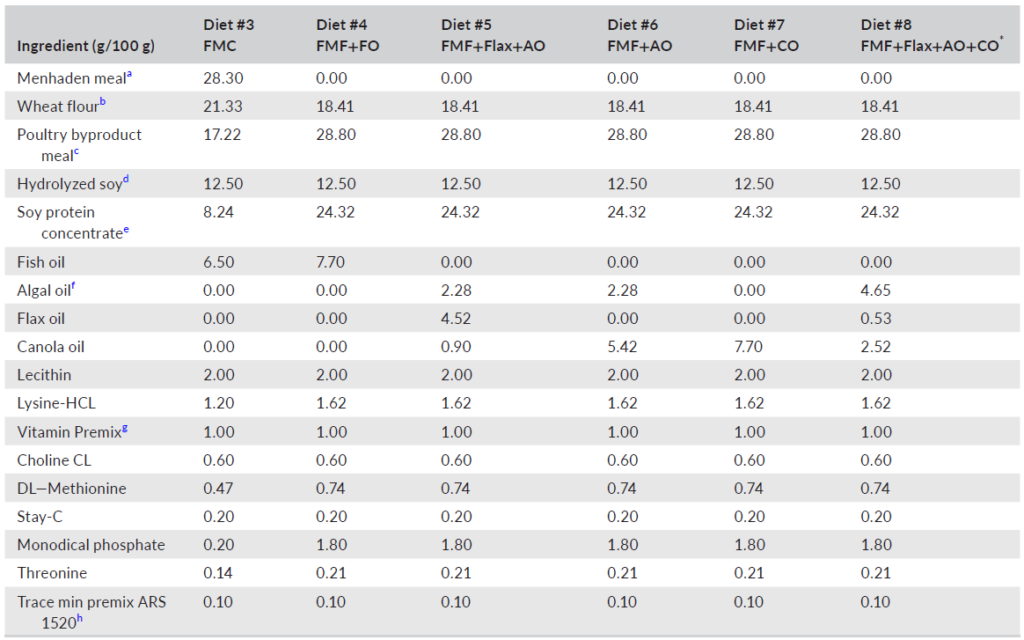
c IDF Inc., California, 70-g/kg crude protein; d MrFeed Pro50FF, Menon Renewable Products Inc., California; e Solae, Pro-Fine VF, Missouri, 656-g/kg crude protein; f Vera Oil, Veramaris®, Nebraska; g USDA ARS, Montana, ARS 702; contributed, per kg diet; vitamin A 9650 IU; vitamin D 6600 IU; vitamin E 132 IU; vitamin K3 1.1 gm: thiamin mononitrate 9.1 mg; riboflavin 9.6 mg; pyridoxine hydrochloride 13.7 mg; pantothenate DL-calcium 46.5 mg; cyanocobalamin 0.03 mg; nicotinic acid 21.8 mg; biotin 0.34 mg; folic acid 2.5 mg; inositol 600 mg; h USDA ARS, Montana, ARS 1520, contributed in mg/kg of diet; copper 10; iodine 6; iron 90; manganese 17; zinc 30; *DHA balanced.
consume as much diet as they wanted for approximately 5 min in the morning and evening. This approach allowed the LMB to be fed at a rate close to apparent satiation without overfeeding and enabled accurate calculation of feed conversion ratio. Fish in each aquarium were collectively weighed every 3 weeks and at week 10 during trial termination, to monitor weight gain and feed conversion ratio. At the 6-week weighing, four fish of average weight were removed from each aquarium to reduce biomass.
The RAS maintained water quality conditions well within acceptable levels for LMB (Tidwell et al., 2018). Water quality parameters (±SD) throughout the trial were as follows: temperature: 27.8 ± 0.5°C, dissolved oxygen: 5.7 ± 0.6 mg/L, total ammonia nitrogen: 0.074 ± 0.037 mg/L, total nitrite nitrogen: 0.04 ± 0.03 mg/L, pH: 7.6 ± 0.1 and salinity: 1.2 ± 0.4 g/L, 15.2 g.
At trial end (week 10), production parameters such as per cent weight gain ([g final weight −initial weight/g initial weight] ×100), feed conversion ratio (g dry feed offered/g weight gain) and survival (%) were recorded for fish in each aquarium. Three fish per aquarium (n = 9 per diet) were randomly selected and euthanized with an overdose of tricaine methane sulphonate (MS-222) at 300 mg/L (Topic Popovic et al., 2012) to measure the following indices: hepatosomatic index (HSI) (100 × g liver weight/g body weight), intraperitoneal fat (IPF) ratio (100 × g IPF weight/g body weight), fillet yield (g fillet weight/100 g body weight) and Fulton’s condition factor (CF) (g fish weight/cm3 fish length). Three fish per aquarium also were homogenized as a composite sample and analyzed for proximate composition using the following established methods: the Dumas protocol for crude protein (6.25 × N; AOAC, 2005), chloroform:- methanol extraction for crude lipid (Folch et al., 1957) and heating samples at 650°C in the muffle furnace for 3 h for ash (AOAC, 1990). Protein efficiency ratio (PER) ([weight gain (g)/protein intake (g)] × 100) also was computed (Rosales et al., 2017).
TABLE 2 Proximate composition of experimental and commercial diets fed to largemouth bass (100 g of diet on a dry-matter basis)
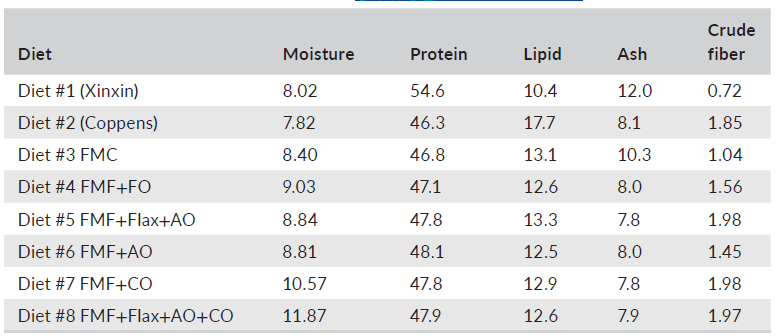
Abbreviations: AO, algal oil; CO, canola oil; FMC, fishmeal control; FMF, fishmeal-free; FO, fish oil.
TABLE 3 Fatty acid composition (%) of alternative oils used in experimental diets fed to largemouth bass
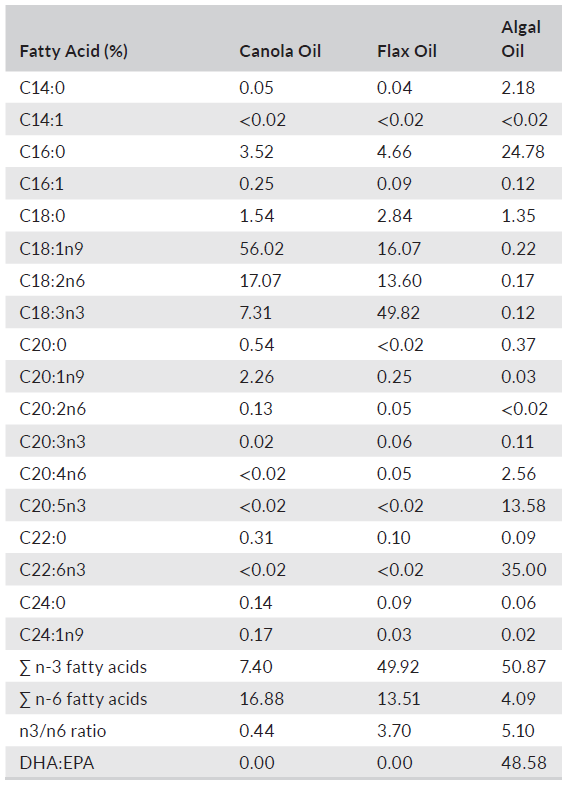
Following sampling at week 10, the remaining fish from three replicate aquaria were consolidated into two aquaria and fed their experimental diets for an additional 2 weeks. During this period, at 3-day intervals, faecal samples were collected from all fish in each aquarium approximately 5 h after feeding using the well-established stripping technique. Fish were netted out of the tank, and faecal contents were collected within each experimental unit by manually stripping the lower intestine into sterilized pans cleaned with 70% ethanol before collection. After faecal sample collection, fish were immediately placed in an oxygen-rich environment to recover then returned to their respective aquaria. Samples collected from each aquarium were dried at 60°C for 24 h, pooled over time as one composite sample (averaging ~1 g of dried faeces) per aquarium, finely ground with mortar and pestle, placed into sterile bags and stored at −20°C until analyzed. Coefficients of digestibility for the various diets were computed after analyzing diets and faecal samples for organic matter (dry matter –ash), crude protein and crude lipid according to the procedures described above for whole-body tissues. In addition, gross energy was determined with an adiabatic calorimetry while yttrium and phosphorus were analyzed via inductively coupled plasma spectroscopy (AOAC, 2005). The digestibility coefficients for each diet were computed according to the following formula: ADC = 100 × (1 − (%yttrium in diets/%yttrium in faeces) × (%nutrient
in faeces/%nutrient in diets)).
2.3 | Statistical analysis
Response variables were subjected either to the Student’s t-test to compare fishmeal replacement (diets 3 and 4) or to one-way analysis of variance (ANOVA) based on a completely random design using the general linear model of the Statistical Analysis System to compare fish oil replacement (diets 5 to 8). Data were analyzed using JMP software (V 14.1, SAS institute, California, USA). If significant (p < 0.05) differences were detected, data were further subjected to Tukey HSD analysis for comparison of mean values. The homogeneity of variance was analyzed using the Brown-Forsythe test (1974).
3 | RESULTS
The LMB juveniles grew rapidly during the feeding trial with individual fish weight of all dietary treatments averaging 68.5 g after 10 weeks of feeding. Fish fed all diets except the Coppens reference diet increased their initial weight by over 300%, representing an over 4.5-fold increase of initial weight (Table 5). Largemouth bass fed the Coppens diet had lower (p < 0.05) weight gain than all
TABLE 4 Fatty acid analysis of complete diets including commercial and experimental feeds fed to largemouth bass (Micropterus salmoides)
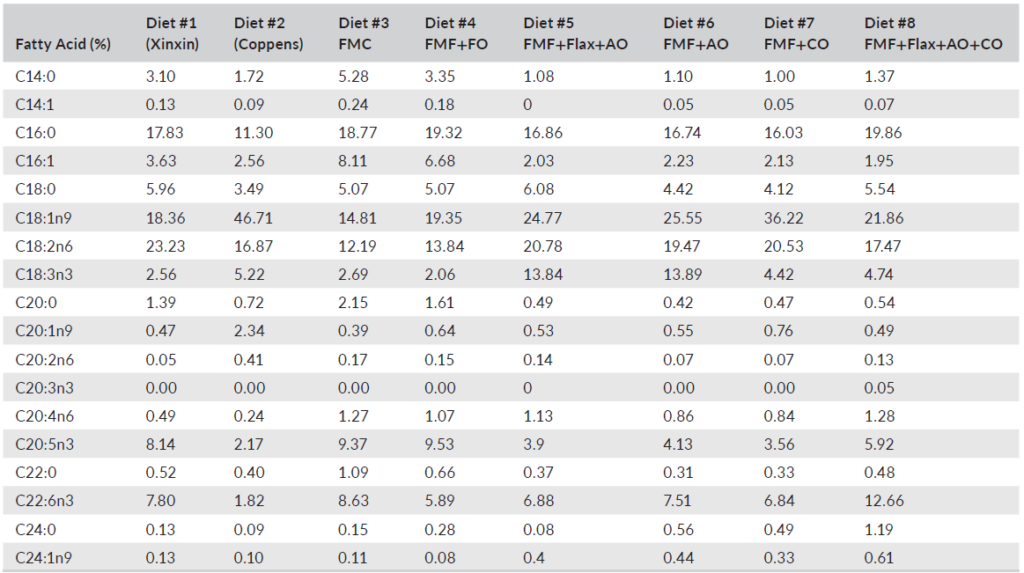
fish fed all other diets except for diet 3. Diets 4, 6 and 8 supported weight gain similar to that of fish fed the Xinxin diet, although diets 3, 5 and 7 also were statistically similar. The various diets yielded PER values ranging from 2.14 to 2.34 with no statistical differences (Table 5) and survival above 98%. Fish fed the Coppens diet had the lowest survival with 11 mortalities observed for an average of 81.7% throughout the trial. The Xinxin reference feed and diets 3 and 8 experienced single mortalities (Table 5) while all other groups had 100% survival (Table 5). All experimental diets supported excellent feed conversion, returning values <1.0, with no differences (p > 0.05) between them and the commercial reference diets (Table 5).
The various body condition indices including intraperitoneal fat ratio, fillet yield and Fulton condition factor were not affected by dietary treatment. However, HSI illustrated considerable variation among the diets. Largemouth bass fed the Xinxin reference diet, and diet 7 had the lowest HSI values at 2.6% (Table 5), with all other diets resulting in HSI values ranging from 3.0 to 4.1. The potential reasons for these differences were not readily apparent. Differences (p < 0.05) in whole-body protein, lipid and ash also were detected in fish fed the various diets (Table 6). The most pronounced variation was the lower protein and higher lipid content of bass fed the Coppens diet compared to fish fed all the other feeds. This was likely due to the higher level of lipid in the Coppens diet at 17.7% compared to 10.4% for the Xinxin feed and less than 13.3% for the experimental feeds. Apparent digestibility coefficients for organic matter, crude protein, crude lipid and gross energy, as well as phosphorus availability, were all relatively high and generally similar among the various experimental diets (Table 7). However, Diet 8 had the highest digestibility coefficient for organic matter, which was significantly higher than that of Diets 6 and 7. Similarly, Diet 8 had the highest crude protein digestibility, which differed from Diets 1 through 3.
Diet had a significant impact on LMB whole-body fatty acid profiles (Table 8). Noteworthy was Diet 3, which incorporated FM and FO, expressed highest levels of myristic acid (C14:0), palmitoleic acid (C16:1) and eicosapentaenoic acid (C20:5n-3; EPA). Fish-fed diets that included flax oil expressed highest amounts of α-linoleic acid (C18:3n-3), while those provided with the canola oil-based diet (Diet 7) and the Coppens feed deposited the highest proportions of linoleic acid (C18:2n-6). Diets formulated using algal oil had whole-body levels of docosahexaenoic acid (C22:6n-3; DHA), as high or higher than observed in fish fed the diets supplemented with FO (FMC and FMF+FO). Overall, n-3 isomers were highest (p < 0.01) in the FMC (Diet 3) and flax + algal oil (Diet 5) fed fish >flax + algal +canola oil (Diet 8; p < 0.01) > Diets 2, 4 and 6 (p < 0.01) > Diets 1 and 7 (p < 0.01). Total n-6 isomers also differed between diets with those containing flax and/or canola oil yielding highest levels (p < 0.01; Table 8).
TABLE 5 Production performance, condition indexes and survival of largemouth bass fed the experimental diets for 70 days
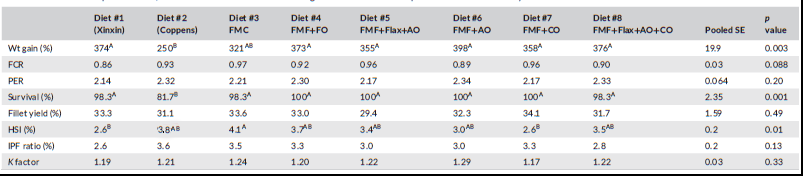
Abbreviations: AO, algal oil; CO, canola oil; FMC, fishmeal control; FMF, fishmeal-free; FO, fish oil.
4 | DISCUSSION
There remains some debate over the optimal dietary protein and lipid requirements for juvenile LMB. Some studies report acceptable growth using diets containing ≤45% crude protein (Anderson et al., 1981; Portz et al., 2001), whereas others set the requirement between 47 and 51% (Cai et al., 2020; Chen et al., 2012; Csargo et al., 2013; Huang et al., 2017). These reported discrepancies may reflect differences in strains of LMB, variations in husbandry (feeds, system, water quality, etc.), trial duration, age and other factors. Given appropriate levels of dietary protein, however, recommended lipid levels for LMB fall in the range of 11.5–14.0% (Bright et al., 2005; Chen et al., 2012; Huang et al., 2017). Accordingly, all experimental diets used in the present trial expressed protein and lipid levels that were within published target ranges and ratios for the species although lower than that found in current commercial feeds.
Fish fed all experimental feeds had similar weight gain and survival values matching those of LMB fed the Xinxin feed. These results are remarkable because the Xinxin feed contained 15% higher and 20% lower levels of crude protein and lipid, respectively, than the experimental diets. The use of blended proteins, as illustrated here, combined with results from other successful studies with LMB, where major proportions of dietary FM were replaced (McLean, Fredriksen, Alfrey, Craig, et al., 2020; McLean, Fredriksen, Alfrey, Tlusty, et al., 2020; Ren et al., 2018; Subhadra et al., 2006b; Tidwell et al., 2005; Wang et al., 2018; Yi et al., 2020;), provides strong and realistic support to suggest that future LMB grower feeds can be formulated devoid of FM. LMB have been reported to respond positively to increasing levels of dietary lipid, with fish expressing increased weight gain, improved feed efficiency and protein-sparing action (Anderson et al., 1981; Guo et al., 2019; Li et al., 2020a). While it is possible that the higher lipid levels of experimental diets provided similar benefits to enable performance parity with the high-protein Xinxin diet, the compromised growth and survival of LMB fed the Coppens feed, combined with equivalent PER and FCR values across all groups, would challenge such an assumption. The results of the described trial thus support the conclusions of Huang et al. (2017), who suggested that lipid enrichment of LMB feeds has no, or only limited protein-sparing action. An alternative explanation for the poorer performance accrued by the Coppens-fed fish may be the presence of additional dietary ingredients. For example, increasing levels of starch reduces weight gain and protein retention of juvenile LMB (Li et al., 2020b).
It is worthy to note that total n-3 and n-6 levels differed noticeably among the alternative oils employed, with canola oil presenting lowest n-3 to n-6 ratios (0.4) and the algal oil expressing highest (5.1) ratios. In other trials, dietary n-3 to n-6 ratios of 0.1 have been reported as sufficient for growth, and although the precise fatty acid requirements of LMB remain unknown, it is generally suggested that any dietary needs for EPA/DHA are low and that demands can be satisfied through hepatic conversion of linolenic acid (Yadav et al., 2020). The lack of effect of the three alternative oils, and their blends, on growth, survival and feed conversion, which support the findings of others (Subhadra et al., 2006a; Tidwell et al., 2007; Yun et al., 2013; Zhang, Li, et al., 2019, Zhang, Tan, et al., 2019), illustrates the feasibility of totally excluding FO from LMB diets. It should nevertheless be noted that dietary alternative proteins and
TABLE6 Whole-body proximate composition of largemouth bass fed the experimental diets for 10 weeks (% of fresh weight)
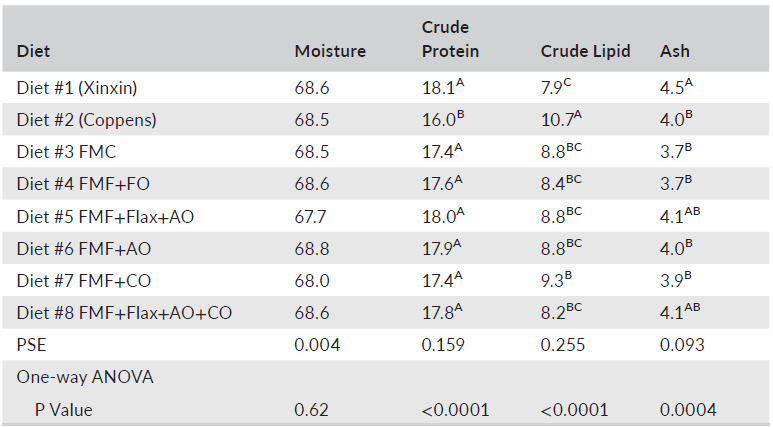
TABLE 7 Percent apparent digestibility coefficients (ADC) and phosphorus (P) availability of eight experimental diets fed to largemouth bass
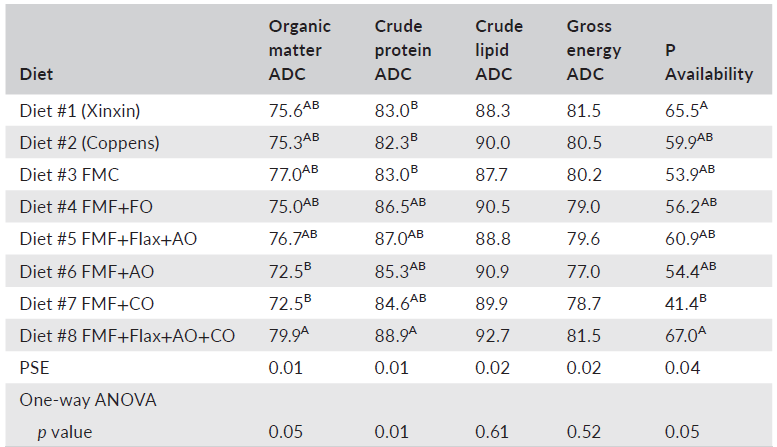
oils have been associated with significant changes in various physiological control processes. For example, increased oxygen consumption, elevated pepsin levels, disturbance in various hepatic enzymes, changes in hepatic gene expression profiles, negative impacts on LMB immunity and, inter alia, histopathological effects upon the gut and changes therein to the microbiome have all been detected (e.g., Habte-Tsion et al., 2020; He et al., 2020; Shi et al., 2019; Subhadra et al., 2006a; Zhang, Li, et al., 2019, Zhang, Tan, et al., 2019; Zhou et al., 2018; Zhu et al., 2019). Whether these and other adjustments are consequential to animal welfare and production remains unresolved, but each certainly deserves further examination.
Most trials with LMB and alternative proteins and oils report changes in whole-body and fillet composition. However, these effects are inconsistent and appear to vary not only with protein/oil source, but also with feed intake, age (perhaps reflecting more rapid growth rates of younger fish) and trial length (Subhadra et al., 2006a,2006b). Whole-body compositional analyses tend to reflect that of the dietary formulation. Higher dietary lipid is also often associated with elevated hepatosomatic indices (HSI), and although differences were observed among feed groups in the current trial, these did not reflect on diet composition. While fish from the diet supplemented only with canola oil (Diet 7) returned lowest values for HSI, which may indicate differences in energy partitioning, the general similarity in HSI, equivalence in intraperitoneal fat ratios and condition factors, and lack of effect of alternate oils on glycogen and lipid accumulation in other studies with LMB (McLean, Fredriksen, Alfrey, Craig, et al., 2020) imply equal utilization of the vegetable and algal oils.
TABLE 8 Fatty acid profile of largemouth bass whole-body samples from fish fed the experimental diets for 10 weeks
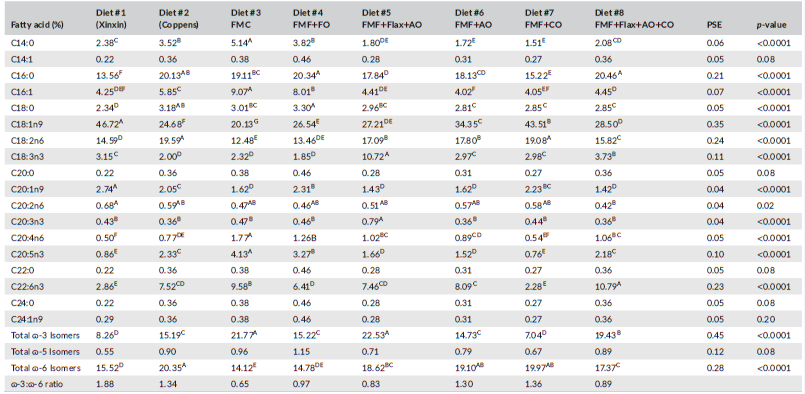
Abbreviations: AO, algal oil; CO, canola oil; FMC, fishmeal control; FMF, fishmeal-free; FO, fish oil.
It is generally reported that fatty acid profiles of fish commonly reflect those of their diet (Xu et al., 2020), and the results presented here are consistent with this paradigm and support the findings of other studies with LMB in which FO was entirely replaced by alternatives (Chen et al., 2020; Subhadra et al., 2006a; Tidwell et al., 2007; Yun et al., 2013). Ratios of total n-3 to n-6 fatty acids varied both with diet and flesh content, lowest ratios being discerned in fish-fed experimental diets 6 and 7. LMB fed diets in which FO was replaced by corn, sunflower, soybean or palm oil have been reported to exhibit significant reductions in fillet DHA and EPA relative to FO-based diets (Chen et al., 2020; Laporte & Trushenski, 2011; Tidwell et al., 2007; Yun et al., 2013). Observations from the present study, therefore, might be considered remarkable although perhaps not unanticipated, given that most diets were all supplemented with DHA-EPA enhanced algal oil at 2.28–4.65%, and DHA is selectively retained (Xu et al., 2020; Yun et al., 2013). Nonetheless, the fish-fed experimental feeds expressed higher DHA:EPA ratios than those receiving the FMC feeds.
A general impact of vegetable oils on fish fillets has decreased juiciness and higher levels of dietary linoleic acid (18:2n-6). For example, a diet rich in 18:2 n-6 has been reported to discernably increase fillet whiteness, tenderness, juiciness and sweetness (Ti et al., 2019). Likewise, canola oil is without effect on salmon texture, although soybean oil may cause flesh to become softer (Rosenlund et al., 2010). Yang and colleagues (2020) reported that rapeseed, palm, soybean and linseed oils increased the cohesiveness, gumminess and springiness of grass carp muscle, while decreasing fillet hardness. In general, total replacement of FO with individual vegetable oil or blends causes changes in aroma and flavour (Rosenlund et al., 2010). When FO was replaced using soybean oil, this was found to be inconsequential to the organoleptic characteristics of LMB fillets (McLean, Fredriksen, Alfrey, Tlusty, et al., 2020). Nevertheless, further trials on the impact of different oil types on organolepti attributes, storage stability, processing, cooking and other quality-related issues, are clearly desirable. Were problems with product quality to be distinguished, a potential way to avoid negative influences that vegetable oils may have could be with the use of finishing feeds, where, for the final few weeks of production, diets with FO are fed in attempts to restore conventional profiles (Xu et al., 2020). Likewise, the current and other studies demonstrate the feasibility of developing designer fish using feeds conceived to influence product quality (Cotter et al., 2008; Rasmussen et al., 2000). For example, increasing dietary algal oil to beneficially impact fish muscle DHA levels might be employed to increase product purchases of health-conscious consumers.
Like FM, alternatives to FO are generally less expensive. For example, while the typical price of ‘aquaculture grade’ FO in 2020 hovered around $1900 per tonne (Craze, 2020), and for those containing high levels of omega-3s (EPA/DHA), $2800 (Bacon, 2020), substitutes, such as canola and soybean oils, were around US$ 970 per tonne (Index Mundi, 2020). Another undesirable feature of FO is that, in its crude form, it may contain a variety of contaminants including polychlorinated biphenyls (PCBs), polychlorinated dibenzo-p- dioxins (PCDDs), furans (PCDFs), polybrominated diphenyl ethers (PBDEs) and organochlorinated pesticides (OCPs; Jacobs et al., 2002; Li et al., 2018; Nøstbakken et al., 2021). These toxins necessitate refining steps, such as filtration over activated carbon, enzymatic treatment, supercritical CO2 extraction or steam distillation, for decontamination (Chang et al., 1989; Merkle et al., 2017; Wang et al., 2019), which adds to cost of production. On the other hand, when vegetable or algal oils are used to replace fish oil, fillet levels of persistent organic pollutants are greatly reduced (Bell et al., 2005,2012; Berntssen et al., 2005; Ginés et al., 2018). The algal oil used herein was derived from Schizochytrium sp. produced by controlled heterotrophic fermentation, using dextrose as the carbon source. These production methods are generally considered contaminant-free, resulting in negligible to zero levels of toxicants when compared against the fillets from FO-fed fish (Sprague et al., 2017). That is not to say that vegetable and algal oils are void of contaminants, because levels of some pollutants, such as poly-aromatic hydrocarbons, which are insoluble in water, may be present (Berntssen et al., 2010; Glencross et al., 2020).
Based on the results from the current study, total replacement of dietary FM/FO in largemouth bass feeds is clearly a realistic and economically viable proposition. Even so, further production-length research is required to assess the impact that such diets may have on the overall health and welfare of farmed animals. The effect that dietary changes may present to various quality attributes that influence wholesale, retail and consumer purchasing decisions also need greater examination. Clearly, however, alternate protein/oil combinations provide final products that are more secure in terms of food safety and likely more acceptable to the discerning consumer. The use of such nutrients acts to bridge gaps between the supply and demand for FM/FO and serve global sustainability initiatives.
ACKNOWLEDGEMENTS
The authors are indebted to the Future of Fish Feed (F3) for their financial, research and technical support. Technical support of Brian Ray and graduate students at Texas A&M University with the execution of the feeding trial and laboratory analyses is also gratefully acknowledged.
CONFLI CT OF INTEREST
The authors declare no conflict of interest.
AUTHOR CONTRIBUTIONS
F.T.B., D.M.G., E.M. and K.B.A. designed the study; D.M.G. conducted the study; E.M. analyzed the data; D.M.B., K.B.A. and E.M. wrote the manuscript.
DATA AVAILABILITY STATEMENT
The data that support the findings of this study are freely available on request from the corresponding author.
ORCID
Ewen McLean https://orcid.org/0000-0002-2832-7502
Delbert M. Gatlin III https://orcid.org/0000-0003-2534-6422
REFERENCES
Anderson, R. J., Kienholz, E. W., & Flickinger, S. A. (1981). Protein requirements of smallmouth bass and largemouth bass. Journal of Nutrition, 111, 1085–1097.
Anonymous (2016). La creciente invasion china desafía a la pesca gallega. Faro de Vigo 13th November 2016.
AOAC (1990). Official methods of analysis. Association of Official Analytical Chemists, Arlington.
AOAC (2005). Official methods of analysis. Association of Official Analytical Chemists, Arlington.
Bacon, W. S. (2020, December 26). Fishmeal comments and prices.
Hammersmith Marketing Ltd, weekly report. https://hamme rsmit hltd.blogs pot.com/
Bai, J., & Li, S. (2018). Development of largemouth bass (Micropterus salmoides) culture. In J. F. Gui, Q. Tang, Z. Li, J. Liu, & S. S. De Silva (Eds.), Aquaculture in China: Success stories and modern trends (pp.
421–429).
John Wiley & Sons.
Bell, J. G., Dick, J. R., Strachan, F., Guy, D. R., Berntssen, M. H. G., &
Sprague, M. (2012). Complete replacement of fish oil with a blend of vegetable oils affects dioxin, dioxin-like polychlorinated biphenyls (PCBs) and polybrominated diphenyl ethers (PBDEs) in 3 Atlantic salmon (Salmo salar) families differing in flesh adiposity.
Aquaculture, 324–325, 118–126.
Bell, J. G., McGhee, F., Dick, J. R., & Tocher, D. R. (2005). Dioxin and dioxin-like polychlorinated biphenyls (PCBs) in Scottish farmed salmon (Salmo salar): Effects of replacement of dietary marine fish oil with vegetable oils. Aquaculture, 243, 305–314.
Berntssen, M. H. G., Julshamn, K., & Lundebye, A.-K.
(2010). Chemical contaminants in aquafeeds and Atlantic salmon (Salmo salar) following the use of traditional-versus alternative feed ingredients.
Chemosphere, 78, 637–346.
Berntssen, M. H. G., Lundebye, A.-K., & Torstensen, B. E. (2005). Reducing the levels of dioxins and dioxin-like PCBs in farmed Atlantic salmon by substitution of fish oil with vegetable oil in the feed. Aquaculture Nutrition, 11, 219–231.
Bright, L. A., Coyle, S. D., & Tidwell, J. H. (2005). Effect of dietary lipid level and protein energy ratio on growth and body composition of largemouth bass Micropterus salmoides. Journal of the World Aquaculture Society, 36, 129–134.
Brown, M. B., & Forsythe, A. B. (1974). Robust tests for the equality of variances. Journal of the American Statistical Association, 69, 364–367.
Cai, J., Zhou, X., Yan, X., Lucente, D., & Lagana, C. (2019). Top 10 species groups in global aquaculture 2017 (p. 12). WAPI Factsheet. FAO.
Cai, L., Qian, X.-Q., & Xie, S.-Q. (2020). Optimal dietary protein concentrations for largemouth bass (Micropterus salmoides) of different sizes (10–500g). Aquaculture International, 28, 831–840.
Castelvetro, V., Corti, A., Bianchi, S., Giacomelli, G., Manariti, A., & Vinciguerra, V. (2020). Microplastics in fish meal: contamination level analyzed by polymer type, including polyester (PET), polyolefins, and polystyrene. Environmental Pollution, 273, 115792.
CFS (2019). China Fishery Statistics Yearbook. Bureau of Fisheries, Ministry of Agriculture, China Agriculture Press.
Chang, S. S., Bao, Y., & Pelura, T. J. (1989). Purification of fish oil. US
Patent 4,874,629 A Chen, N.-S., Xiao, W.-W., Liang, Q.-L., Zhou, H.-Y., Ma, X.-L., & Zhao, M. (2012). Effects of dietary lipid to protein ratios on growth performance, body composition and non-specific immunity of largemouth bass (Micropterus salmoides). Journal of Fisheries China, 36, 1270–1280.
Chen, Y., Sun, Z., Liang, Z., Xie, Y., Su, J., Luo, Q., Zhu, J., Liu, Q., Han, T., & Wang, A. (2020). Effects of dietary fish oil replacement by soybean oil and l-carnitine supplementation on growth performance,
fatty acid composition, lipid metabolism and liver health of juvenile largemouth bass, Micropterus salmoides. Aquaculture, 516, 734596.
Colombo, S. M. (2020). Physiological considerations in shifting carnivorous fishes to plant-based
diets. In T. J. Benfey, A. P. Farrell, & C. J.
Brauner (Eds.), Fish Physiology, 38, Aquaculture (pp. 53–82).
Elsevier. Cotter, P. A., McLean, E., & Craig, S. R. (2008). Designing fish for improved
human health status. Nutrition and Health, 20, 1–9.
Cottrell, R., Blanchard, J., Halpern, B., Metian, M., & Froehlich, H. (2020).
Global adoption of novel aquaculture feeds could substantially reduce
forage fish demand by 2030. Nature Food, 1, 303–308.
Craze, M. (2020, December 28). Fish oil set for price hike in 2020
after poor fishing season in Peru. https://www.under curre ntnews.
com/2020/01/30/fish-oil-set-for-price-hike-in-2020-after-poor-fishing-season-in-peru/
Crona, B., Wassénius, E., Troell, M., Barclay, K., Mallory, T., Fabinyi, M.,
Zhang, W., Lam, V. W. Y., Cao, L., Henriksson, P. J. G., & Eriksson,
H. (2020). China at a crossroads: An analysis of China’s changing seafood production and consumption. One Earth, 3, 32–44.
Csargo, I. J., Brown, M. L., & Ward, M. J. (2013). Evaluation of four commercial grower diets for production of largemouth bass. Journal of Applied Aquaculture, 25, 35–49.
Ding, Q., Chen, Y., Cao, S., & Ye, Y. (2013). Research progress on nutritional requirements of largemouth bass. Aquaculture Feed, 11, 38–43.
Fan, F., Liupu, C., Jian, P., Ningyu, Z., Yijiang, B., Ting, X., Gaohua, Y., Wenjun, M., & Xueyan, D. (2020). The response of juvenile largemouth bass (Micropterus salmoides) to varying protein and carbohydrate levels in extruded feed. International Journal of Fisheries and Aquatic Studies, 8, 466–473.
FAO (2018). The state of world fisheries and aquaculture 2018. Meeting sustainable development goals. FAO.
FAO (2020, December 20). Aquaculture production statistics. www.fao.org/figis
J. Fink-Gremmels
(Ed.) (2012). Animal feed contamination, effects on livestock and food safety. Woodhead Publishing Series in Food Science, Technology and Nutrition, Number 215.
Folch, J., Lees, M., & Stanley, G. H. S. (1957). A simple method for the isolation and purification of total l lipides from animal tissues. Journal of Biological Chemistry, 226, 497–509.
Froehlich, H. E., Jacobsen, N. S., Essington, T. E., Clavelle, T., & Halpern,
B. (2018). Avoiding the ecological limits of forage fish for fed aquaculture.
Nature Sustainability, 1, 298–303.
Gasco, L., Gai, F., Maricchiolo, G., Genovese, L., Ragonese, S., Bottari, T., & Caruso, G. (2018). Feeds for the aquaculture sector: Current situation and alternative sources. Springer International.
Gatlin, D. M., Barrows, F. T., Brown, P., Dabrowski, K., Gaylord, T. G.,
Hardy, R. W., Herman, E., Hu, G., Krogdahl, Å., Nelson, R., Overturf,
K., Rust, M., Sealey, W., Skonberg, D., J Souza, E., Stone, D., Wilson,
R., & Wurtele, E. (2007). Expanding the utilization of sustainable plant products in aquafeeds: A review. Aquaculture Research, 38,551–579.
Ginés, R., Camacho, M., Henríquez-Hernández, L. A., Izquierdo, M., Boada, L. D., Montero, D., Robaina, L., Zumbado, M., & Luzardo, O. P. (2018). Reduction of persistent and semi-persistent organic pollutants in fillets of farmed European seabass (Dicentrarchus labrax) fed low fish oil diets. Science of the Total Environment, 643, 1239–1247.
Glencross, B. D., Baily, J., Berntssen, M. H. G., Hardy, R., MacKenzie, S., & Tocher, D. R. (2020). Risk assessment of the use of alternative animal and plant raw material resources in aquaculture feeds. Reviews in Aquaculture, 12, 703–758.
Gui, L., & Zhang, Q.-Y.
(2018). Disease prevention and control. In J.-F. Gui, Q. Tang, Z. Li, J. Liu, & S. S. De Silva (Eds.), Aquaculture in China: Success stories and modern trends (pp. 577–598).
John Wiley & Sons Ltd.
Guo, J.-J., Zhou, Y., Zhao, H., Chen, W.-Y., Chen, Y.-J., & Lin, S.-M. (2019).
Effect of dietary lipid level on growth, lipid metabolism and oxidative status of largemouth bass, Micropterus salmoides. Aquaculture, 506, 394–400.
Habte-Tsion, H.-M., Kolimadu, G. D., Rossi, W. Jr, Filer, K., & Kumar, V. (2020). Effects of Schizochytrium and micro-minerals on immune, antioxidant, inflammatory and lipid-metabolism status of Micropterus salmoides fed high-and low-fishmeal diets. Scientific Reports, 10, 7457. https://doi.org/10.1038/s4159 8-020-64286-9
Han, D., Shan, X., Zhang, W., Chen, Y., Wang, Q., Li, Z., Zhang, G., Xu, P., Li, J., Xie, S., Mai, K., Tang, Q., & De Silva, S. S. (2018). A revisit to fishmeal usage and associated consequences in Chinese aquaculture.
Reviews in Aquaculture, 10, 493–507.
He, M., Yu, Y., Li, X., Poolsawat, L., Yang, P., Bian, Y., Guo, Z., & Leng, X.
(2020). An evaluation of replacing fish meal with fermented soybean meal in the diets of largemouth bass (Micropterus salmoides): Growth, nutrition utilization and intestinal histology. Aquaculture
Research, 51, 4302–4314.
Huang, D., Wu, Y., Lin, Y., Chen, J., Karrow, N., Ren, X., & Wang, Y. (2017). Dietary protein and lipid requirements for juvenile largemouth bass, Micropterus salmoides. Journal of the World Aquaculture Society, 48, 782–790.
Hussein, G. H. G., Chen, M., Qi, P.-P., Cui, Q.-K., Yu, Y., Hu, W.-H., Tian, Y., Fan, Q.-X., Gao, Z.-X., Feng, M.-W., & Shen, Z.-G. (2020). Aquaculture industry development, annual price analysis and out-of-season
spawning in largemouth bass Micropterus salmoides.
Aquaculture, 519, 734901.
Index Mundi (2020, May 29). www.index mundi.com/commoditie s/?commo dity=soybe an-meal&month s=240&commodity=fish-meal
Jacobs, M. N., Covaci, A., & Schepens, P. (2002). Investigation of selected persistent organic pollutants in farmed Atlantic salmon (Salmo salar), salmon aquaculture feed and fish oil components of the feed.
Environmental Science and Technology, 36, 2797–2805.
Konar, M., Qiu, S., Tougher, B., Vause, J., Tlusty, M., & Fitzsimmons, K.
(2019). Illustrating the hidden economic, social and ecological values of global forage fish resources. Resources, Conservation and Recycling, 151, 104456.
Laporte, J., & Trushenski, J. (2011). Growth performance and tissue fatty acid composition of largemouth bass fed diets containing fish oil or blends of fish oil and soy-derived lipids. North American Journal of Aquaculture, 73, 435–444.
Lei, X., Zhao, R., Geng, Y., Wang, K., Yang, P. O., Chen, D., Huang, X., Zuo, Z., He, C., Chen, Z., Huang, C., Guo, H., & Lai, W. (2020). Nocardia seriolae: a serious threat to the largemouth bass Micropterus salmoides industry in Southwest China. Diseases of Aquatic Organisms, 142, 13–21.
Li, X., Dong, S., Wang, P., Su, X., & Fu, J. (2019). Polychlorinated biphenyls are still alarming persistent organic pollutants in marine-origin animal feed (fishmeal). Chemosphere, 233, 355–362.
Li, X., Dong, S., Zhang, W., Fan, X., Li, Y., Wang, R., & Su, X. (2018). Global occurrence of polybrominated diphenyl ethers and their hydroxylated and methoxylated structural analogues in an important animal
feed (fishmeal). Environmental Pollution, 234, 620–629.
Li, X., Zheng, S., Ma, X., Cheng, K., & Wu, G. (2020a). Effects of dietary protein and lipid levels on the growth performance, feed utilization, and liver histology of largemouth bass (Micropterus salmoides).
Amino Acids, 52, 1043–1061.
Li, X., Zheng, S., Ma, X., Cheng, K., & Wu, G. (2020b). Effects of dietary starch and lipid levels on the protein retention and growth of largemouth bass (Micropterus salmoides). Amino Acids, 52, 999–1016.
Lillebø, A. I., Pita, C., Garcia Rodrigues, J., Ramos, S., & Villasante, S.
(2017). How can marine ecosystem services support the Blue Growth agenda? Marine Policy, 81, 132–142.
McLean, E., Fredriksen, L., Alfrey, K., Craig, S. R., & Barrows, F. T. (2020). Performance of largemouth bass Micropterus salmoides (Lacépède, 1802), fed fishmeal-and fish oil-free diets. International Journal of
Fisheries and Aquatic Studies, 8, 6–10.
McLean, E., Fredriksen, L., Alfrey, K., Tlusty, M. F., & Barrows, F. T. (2020). Growth, integrity, and consumer acceptance of largemouth bass, Micropterus salmoides (Lacépède, 1802), fed marine resource-free diets. International Journal of Fisheries and Aquatic Studies, 8, 365–369.
Merkle, S., Giese, E., Rohn, S., Karl, H., Lehmann, I., Wohltmann, A., &
Fritsche, J. (2017). Impact of fish species and processing technology on minor fish oil components. Food Control, 73B, 1379–1387.
Mo, W. Y., Man, Y. B., & Wong, M. H. (2018). Use of food waste, fish waste and food processing waste for China’s aquaculture industry: Needs and challenge. Science of the Total Environment, 613–614, 635–643.
National Research Council. (2011). Nutrient requirements of fish and shrimp (375p.). National Academies Press.
Naylor, R. L., Hardy, R. W., Bureau, D. P., Chiu, A., Elliott, M., Farrell,
A. P., Forster, I., Gatlin, D. M., Goldburgh, R. J., Hua, K., &
Nichols, P. D. (2009). Feeding aquaculture in an era of finite resources. Proceedings of the National Academy of Sciences, 106, 15103–15110.
Nøstbakken, O. J., Rasinger, J. D., Hannisdal, R., Sanden, M., Frøyland, L., Duinker, A., Frantzen, S., Dahl, L. M., Lundebye, A.-K., & Madsen, L. (2021). Levels of omega 3 fatty acids, vitamin D, dioxins and dioxin-like
PCBs in oily fish; a new perspective on the reporting of nutrient and contaminant data for risk–benefit
assessments of oily seafood.
Environment International, 147, 106322.
Pincinato, R. B. M., Asche, F., & Oglend, A. (2020). Climate change and small pelagic fish price volatility. Climate Change, 161, 591–599.
Portz, L., Cyrino, J. E. P., & Martin, R. C. (2001). Growth and body composition of juvenile largemouth bass Micropterus salmoides in response to dietary protein and energy levels. Aquaculture Nutrition, 7, 247–254.
Rasmussen, R. S., Ostenfeld, T. H., Rønsholdt, B., & McLean, E. (2000). Manipulation of end-product
quality in rainbow trout with finishing diets. Aquaculture Nutrition, 6, 17–23.
Ren, X., Wang, Y., Chen, J.-M., Wu, Y.-B., Huang, D., Jiang, D.-L., & Li, P. (2018). Replacement of fishmeal with a blend of poultry byproduct meal and soybean meal in diets for largemouth bass, Micropterus
salmoides. Journal of the World Aquaculture Society, 49, 155–164.
Rosales, M., Castillo, S., Pohlenz, C., & Gatlin, D. M. III (2017). Evaluation of dried yeast and threonine fermentation biomass as partial fish meal replacements in the diet of red drum Sciaenops ocellatus.
Journal of Animal Feed Science and Technology, 232, 190–197.
Rosenlund, G., Corraze, G., Izquierdo, M., & Torstensen, B. E. (2010). The effects of fish oil replacement on nutritional and organoleptic qualities of farmed fish. In G. M. Turchini, W.-K.
Ng, & D. R. Tocher (Eds.), Fish oil replacement and alternative lipid sources in aquaculture
feeds (pp. 487–522).
CRC Press. Sharma, S. D., Bhagat, A. R., & Parisi, S. (2019). Seasonal variation and biochemical composition of fishmeal. Raw material scarcity and overproduction in the food industry (pp. 1–12).
Springer Briefs in Molecular Science.
Shi, C. M., Zhao, H., Zhai, X. L., Chen, Y.-J., & Lin, S.-M. (2019). Linseed oil can decrease liver fat deposition and improve antioxidant ability of juvenile largemouth bass, Micropterus salmoides. Fish Physiology and
Biochemistry, 45, 1513–1521.
Sprague, M., Betancor, M. B., & Tocher, D. R. (2017). Microbial and genetically engineered oils as replacements for fish oil in aquaculture feeds. Biotechnology Letters, 39, 1599–1609.
Subhadra, B., Lochmann, R., Rawles, S., & Chen, R. (2006a). Effect of dietary lipid source on the growth, tissue composition and hematological parameters of largemouth bass (Micropterus salmonides).
Aquaculture, 255, 210–220. Subhadra, B., Lochmann, R., Rawles, S., & Chen, R. (2006b). Effect of
fish-meal replacement with poultry by-product meal on the growth, tissue composition and hematological parameters of largemouth bass (Micropterus salmoides) fed diets containing different lipids.
Aquaculture, 260, 221–231. Ti, W.-M., Ong, M.-K., & Teoh, C.-Y. (2019). Assessment on the effects
of dietary fatty acids on growth performance, body compositions, plasma lysozyme activity and sensorial quality of juvenile marble goby, Oxyeleotris Marmorata. Aquaculture Reports, 14, 100186.
Tidwell, J. H., Coyle, S., & Bright, L. A. (2007). Effects of different types of dietary lipids on growth and fatty acid composition of largemouth bass. North American Journal of Aquaculture, 69, 257–264.
Tidwell, J., S. Coyle, & L. A. Bright (Eds.) (2018). Largemouth bass aquaculture.
Wellcome Trust.
Tidwell, J. H., Coyle, S. D., Bright, L. A., & Yasharian, D. (2005). Evaluation of plant and animal source proteins for replacement of fish meal in practical diets for the largemouth bass Micropterus salmoides.
Journal of the World Aquaculture Society, 36, 454–463. Topic Popovic, N., Strunjak-Perovic, I., Coz-Rakovac, R., Barisic, J., Jadan, M., Persin Berakovic, A., & Sauerborn Klobucar, R. (2012). Tricaine
methane-sulfonate (MS-222) application in fish anaesthesia.
Journal of Applied Ichthyology, 28, 553–564.
Turchini, G. M., Torstensen, B. E., & Ng, W.-K. (2009). Fish oil replacement in finfish nutrition. Reviews in Aquaculture, 1, 10–57.
Wang, J.-X., Bao, L.-J., Shi, L., Liu, L.-Y., & Zeng, E. Y. (2019). Characterizing PBDEs in fish, poultry, and pig feeds manufactured in China.
Environmental Science and Pollution Research, 26, 6014–6022. https://doi.org/10.1007/s1135 6-018-04057-2
Wang, M., Chen, N., Li, S., Lian, X., & Yan, C. (2018). Study on replacement of fish meal by two plant protein mixtures in diets for largemouth bass (Micropterus salmoides). Journal of Shanghai Fisheries
University, 2018(1), 37–47.
Wang, X.-X. (2009). Studies on fermented soybean meal as a substitute for fishmeal in formulated diets for largemouth bass Micropterus salmoides. Sil Yu Xumu, 2009(1), 58–61.
Wang, Y., Ni, J., Nie, Z., Gao, J., Sun, Y., Shao, N., Li, Q., Hu, J., Xu, P., & Xu, G. (2020). Effects of stocking density on growth, serum parameters, antioxidant status, liver and intestine histology and gene expression of largemouth bass (Micropterus salmoides) farmed in the in-pond raceway system. Aquaculture Research, 51, 5228–5240.
Wu, X., Castillo, S., Rosales, M., Burns, A., Mendoza, M., & Gatlin, D. M. III (2015). Relative use of dietary carbohydrate, non-essential amino acids, and lipids for energy by hybrid striped bass, Morone chrysops
♀ × M. saxatilis ♂. Aquaculture, 435, 116–119.
Xia, Y., Cao, Z., Lin, L., Pan, X., Yao, J., Liu, Y., Yin, W., & Shen, J. (2018). Research progress on main diseases of largemouth bass (Micropterus salmoides). Chinese Journal of Animal Health Inspection, 9, 72–76.
Xu, H., Turchini, G. M., Francis, D. S., Liang, M., Mock, T. S., Rombenso, A., & Ai, Q. (2020). Are fish what they eat? A fatty acid’s perspective. Progress in Lipid Research, 80, 101064.
Yadav, A. K., Rossi, W. Jr, Habte-Tsion, H.-M., & Kumar, V. (2020). Impacts of dietary eicosapentaenoic acid (EPA) and docosahexaenoic acid (DHA) level and ratio on the growth, fatty acids composition and
hepatic-antioxidant status of largemouth bass (Micropterus salmoides).
Aquaculture, 529, 735683.
Yang, G., Jiang, W., Chen, Y., Hu, Y., Zhou, Q., Peng, M. O., Mingren, Q.
U., & Kumar, V. (2020). Effect of oil source on growth performance, antioxidant capacity, fatty acid composition and fillet quality of juvenile grass carp (Ctenopharyngodon idella). Aquaculture Nutrition,
26, 1186–1197.
Yi, S. (2019). Willingness-to-pay for sustainable aquaculture products: Evidence from Korean red seabream aquaculture. Sustainability, 11, 1557. https://doi.org/10.3390/su110 61577
Yi, S., Wang, H. P., Xie, P., Li, X. X., & Yao, H. (2020). Evaluation of growth and gene expression patterns of different strains related to SMD utilization in largemouth bass. Aquaculture, 523, 735214.
Yun, B., Xue, M., Wang, J., Fan, Z.-Y., Wu, X.-F., Zheng, Y.-H., & Qin, Y.-C. (2013). Effects of lipid sources and lipid peroxidation on feed intake, growth, and tissue fatty acid compositions of largemouth bass (Micropterus salmoides). Aquaculture International, 21, 97–110.
Zhang, G. G., Li, X., Cai, X.-B., Zhang, S.-X., Hua, X.-M., Huang, Z.-Y., Li, N.-Y., & Yao, J.-T. (2019). Effect of enzymatic hydrolyzed soybean meal on growth performance, liver function and metabolism of largemouth bass (Micropterus salmoides). Acta Hydrobiologia Sinica, 2019(5), 1001–1012.
Zhang, W., Tan, B., Liu, K., Dong, X., Yang, Q., Chi, S., Liu, H., Zhang, S., & Wang, H. (2019). Effects of different dietary lipids on growth, body composition and lipid metabolism-related enzymes and genes
in juvenile largemouth bass, Micropterus salmoides. Aquaculture Nutrition, 25, 1318–1326.
Zhou, M., Liang, R., Mo, J., Yang, S., Gu, N. A., Wu, Z., Sarath Babu, V., Li, J., Huang, Y., & Lin, L. I. (2018). Effects of brewer’s yeast hydrolysate on the growth performance and the intestinal bacterial diversity of largemouth bass (Micropterus salmoides). Aquaculture, 484, 139–144.
Zhu, W., Lan, H., Tan, Y., Han, Z., Chen, Y., & Gao, L. (2019). Effects of replacing fish meal with compound proteins on the growth performance, body composition and liver biochemical indices of largemouth
bass (Micropterus salmoides). Feed Industry, 2019(16), 35–42.
How to cite this article: McLean, E., Alfrey, K. B., Gatlin, D. M. III, & Barrows, F. T. (2022). Responses of largemouth bass (Micropterus salmoides, Lacépède, 1802) to fishmeal-, and fish oil-free diets. Aquaculture Research, 53, 3036–3047. https://doi.org/10.1111/are.15815