E. McLeana,⁎, F.T. Barrowsb,⁎, S.R. Craigc, Kelly Alfreyd, L. Trane
a Aqua Cognoscenti, West Columbia, SC, USA
b Aquatic Feed Technologies LLC, Bozeman, MT, USA
c Optimal Aquafeeds, Brookings, SD, USA
d Anthropocene Institute, Palo Alto, CA, USA
e ShrimpVet Laboratory, 307 Nong Lam University, Ho Chi Minh City 720371, Viet Nam
A B S T R A C T
Corresponding to projected global population growth, the world’s food production systems must likewise enlarge substantially if current levels of dietary protein intake are to be maintained. The anticipated growth curve for global aquaculture, and especially for shrimp farming, which is forecast to double production by 2030, will inevitably result in shortfalls in currently favored dietary ingredients: fishmeal (FM) and fish oil (FO). Servicing the projected FM-FO shortfall with marine products would be a formidable task as economic, social, moral, safety and environmental issues have forced the feed sector to examine the use of alternative, non-marine, ingredients. Herein, various alternative protein and lipid sources were evaluated as substitutes for FM-FO in Pacific whiteleg shrimp diets. Growth trials were coupled to challenges with viral and bacterial pathogens to assess potential benefit or disadvantage of ingredient switching. No differences (P > .05) were observed between control and test diets regarding growth or survival, Average Daily Gain (g d−1) or Specific Growth Rate (% d−1) over a 56-day period. Moreover, response to post-feeding trial challenge with EMS/AHPND or WSSV also did not differ between diets (P > .05). However, there was a difference in size/age responsiveness to bacterial challenge, with younger animals succumbing to infection more rapidly (P ≤ .035). Similar responses were observed for shrimp exposed to WSSV over the first 5-days post-challenge (P ≤ .02) after which survival rates equalized. Overall, the results support the sustained belief that FM-FO constituents of Pacific whiteleg shrimp diets can be totally replaced by non-marine ingredients that are nutritionally equivalent. Improvement in responsiveness to substitute proteins and oils might be further gained with more judicious blending of ingredients and use of other additives. Such manipulations could also result in less expensive sustainable feeds.
- Introduction
Since the turn of the century, Pacific whiteleg shrimp, Penaeus vannamei (Boone, 1931), has dominated the shrimp farming sector (FAO, 2018a, 2018b). Global annual production of almost 4.5 million tons in 2017 made P. vannamei the 6th most cultivated of aquatic species, representing over 80% of all farmed shrimp and more than half of all cultured crustaceans, with a value exceeding $26 billion (Fig. 1a; Cai et al., 2019). The preeminent position of whiteleg shrimp, was gained over that of the previously dominant tiger prawn, P. monodon (Fabricius, 1798), for a variety of reasons (Kumar and Engle, 2016). Principally, diseases blighted production and value of the tiger prawn industry (Thitamadee et al., 2016; Shinn et al., 2018). The sector counteracted these losses using specific pathogen free (SPF) and similar, disease-free or resistant (SPR, SPT, USS, HHS; see Alday-Sanz et al.,
2018), whiteleg stocks. From 2000 to 2017 whiteleg shrimp production expanded over 28.8-fold (Fig. 1a), while that for tiger prawn increased by 17%, from around 631,000 to 740,000 t (FAO, 2018a). All forecasts predict that the shrimp aquaculture sector will continue to grow, albeit at a lower trajectory than seen historically. Even given SPF stocks and enhanced biosecurity protocols the industry remains vulnerable to diseases and massive losses have been experienced across Asia (Shinn et al., 2018) and elsewhere (Karunasagar and Karunasagar, 2018; Morales-Covarrubias et al., 2018; Salazar et al., 2010), due to Early
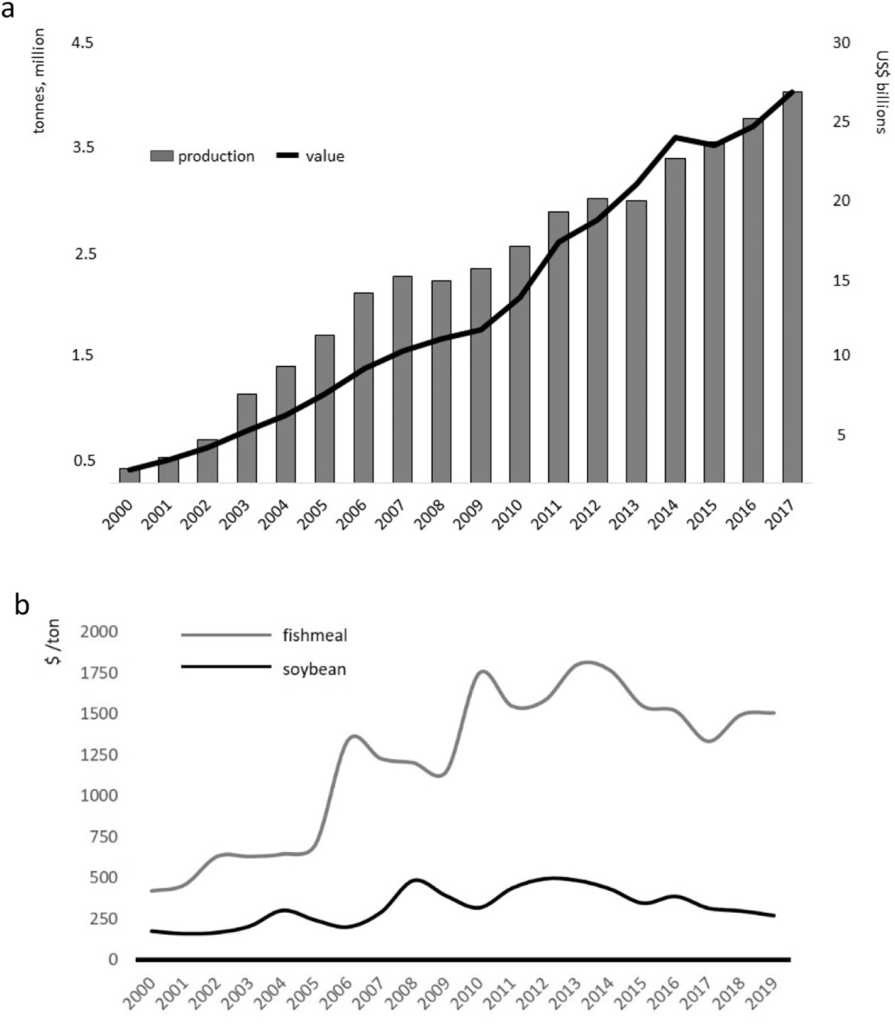
Mortality Syndrome/Acute Hepatopancreatic Necrosis Disease (EMS/AHPND) and White Spot Syndrome Virus (WSSV). EMS/AHPND is a result of infection by pathogenic Vibrio parahaemolyticus (VpAHPND+), and perhaps other strains (Muthukrishnan et al., 2019), that contain a ~ 70-kb plasmid (pVA1) encoding homologues of the Photorhabdus insect-related binary toxin (PirABVp). The molecular and pathogenic characteristics of WSSV, caused by the baculoviral complex of the genus Whispovirus, family Nimaviridae, and its global dissemination, have been ably reviewed elsewhere (Escobedo-Bonilla et al., 2008; Li et al., 2019; Oakey et al., 2019).
Together with the introduction of SPF stocks, uninterrupted expansion of shrimp aquaculture production has been enabled by technological developments in feed manufacture and continued growth of the industry will be contingent upon the feed sector operating more sustainably and reducing pressure on traditional marine ingredients. Commercial feed manufacturers are still heavily reliant on fishmeal (FM) to supply the nutritional requirements of farmed aquatic organisms, and approximately 75% of annual FM (Hulefeld et al., 2018) and fish oil (FO; Auchterlonie, 2018) production is diverted to the aquaculture feed sector. While shrimp represent approximately 5.6% of global aquaculture output (FAO, 2018a), they account for 31% of the annual supply of FM (Malcorps et al., 2019); which is compounded into about 6.2 million tonnes, or 16% of global aquaculture feed production (Tacon and Metian, 2015). The marine ingredient inequity between shrimp and other aquacultured organisms’ feeds is tolerated mainly due to shrimp performance, value and demand. Marine ingredients provide an outstanding balance of essential amino acids (EAA) and fatty acids (EFA), and are an excellent source of vitamins and minerals, in a highly palatable package. However, the rising cost of FM/FO-based formulations, stagnant supply of raw materials, sociological, moral and sustainability arguments, together with feed safety issues, have compelled the aquafeed sector, in general, to examine not only the use of alternative feed ingredients, which are more defensible fiscally and environmentally (Naylor et al., 2009), but also other zootechnical improvements that enhance production efficiency and sustainability. While not a simple undertaking, the substitution of FM-FO, represents the most urgent issue facing the industry today. This is especially critical in marine species feeds, particularly in shrimp aquaculture.
Since 2000, there has been a steady and remarkable decay in the amount of FM employed by shrimp feed manufacturers. Indeed, over the first fifteen years of this millennium, FM inputs into shrimp feeds had been halved, while the use of FO fell below 2% inclusion rates (Salin et al., 2018; Malcorps et al., 2019). These declines are likely to continue until perhaps a 6% inclusion is achieved for FM and > 0.3% for FO by 2025 (Salin et al., 2018). Sustainable commodities that are commonly evaluated as replacements for FM-FO are most often of plant origin, especially grains, pulses and oilseeds (Gasco et al., 2018). However, the use of many alternative plant proteins is problematic because they conceal anti-nutritional factors (ANFs) and or express low biological value due to EAA deficiencies/imbalances, reduced palatability and or poor digestibility (Gatlin et al., 2007). In addition, some alternatives to FM-FO may have negative consequences to animal welfare, including changes to gut and hepatic integrity, and reduced antioxidant and immune status (Lin and Mui, 2017; Sitjà-Bobadilla et al., 2005). These impediments may increase risks of disease. Various feed supplements have been shown to increase growth and improve feed conversion ratios (FCR; Jamal et al., 2019; Toledo et al., 2019) and it is now well-established that dietary ingredients may also beneficially influence the gut microbiome, contributing significantly to shrimp health and welfare (Luis-Villasenor et al., 2013; Dawood et al., 2017; Hossain et al., 2019). The exchange of FM with alternative proteins must, therefore, deliver favorable response in terms of growth and animal health. Ultimately, the provision of elite diets will rely not only on complete knowledge of dietary requirements, but also on the digestibility and integrity of feed ingredients.
In recent years, novel fermentation and processing methods have been utilized to make plant-based dietary replacements more nutritionally complete, efficient and palatable. Of the great variety of alternative plant and animal proteins tested for shrimp feeds, soybean (SB) appears to offer the greatest potential due to its availability and cost (Fig. 1b), acceptable amino acid profiles (Fig. 7) and handling and processing characteristics. Moreover, technological advances in SB processing, such as fermentation, new methods of aqueous extraction and application of novel buffers and enzymes that influence the extraction process and speed of separations (Campbell, 2010), have resulted in improved product lines that express different characteristics, such as comminution and amino acid digestibility (Al-Loman et al., 2018; Mukherjee et al., 2016). These novel make-ups, when presented as blends with different bean varieties, may offer nutritional and other advantages to cultured aquatic animals (see Lagos and Stein, 2017). Based on this, our study aims to eliminate the use of dietary FM-FO using, in the main, SB and poultry by-product meals. The impact of alternative dietary proteins on disease resistance was also assessed with reference to EMS/AHPND and WSSV challenges of two size classes of shrimp.
- Materials and methods
2.1. Experimental animals and husbandry
SPF L. vannamei employed in both growth and challenge trials were purchased from the ShrimpVet Hatchery, Ninh Thuan Province, Vietnam. Originally derived from Shrimp Improvement Systems, Kailua-Kona, Hawai’i (Moss et al., 2012), the broodstock and larvae were evaluated by PCR and confirmed negative (OIE, 2019), for each of EMS/AHPND, WSSV, Taura Syndrome Virus (TSV), Infectious Myonecrosis Virus (IMNV) and Enterocytozoon hepatopenaie (EHP; Tang et al., 2015). Post-larvae (PL) 1 – PL 10 were maintained in 320 L indoor cylindrical tanks supplied with 250 L brackish (20‰) water at 28.14 ± 0.75 °C, pH 7.87 ± 0.05, and DO2 6.82 ± 0.01 mg L−1, under strict biosecurity for 30–45 days, until animals attained a predicted average weight of 0.5–2.5 g. Each holding tank was covered and equipped with an individual biofilter and aeration system. During this transitional period animals were kept on a 12 h:12 h light-dark photoperiod using fluorescent lighting and fed a commercial diet (Grobest Holdings Ltd., Tao-Yuan City, Taiwan), 4 x daily to apparent satiation.
2.2. Diet preparation and feeding
Five isolipidic and isonitrogenous diets were formulated (Table 1) and prepared in-house. The positive FM control diet (FMC), contained solvent-extracted menhaden FM (Daybrook Fisheries, New Orleans, Louisiana, USA) at 27% dry weight. FM was swapped out by poultry meal (PM, Tyson Foods Inc., River Valley Animal Foods, Texarkana,
Table 1
Composition of fishmeal control (FMC) and experimental diets employed to evaluate the efficiency of various alternate blended proteins in diets for Pacific whiteleg shrimp. PBM = poultry by-product meal, FSM = fermented soy meal, F2 = FM-free, and CSF= negative soy control. Formulation values are g/100 g diet as fed. In addition to the experimental and FMC diets, a commercial shrimp feed (CSF), marketed as a functional diet (Grobest™; GRO), and widely employed by the shrimp farming sector in Vietnam, was incorporated into the trial as an additional positive control.
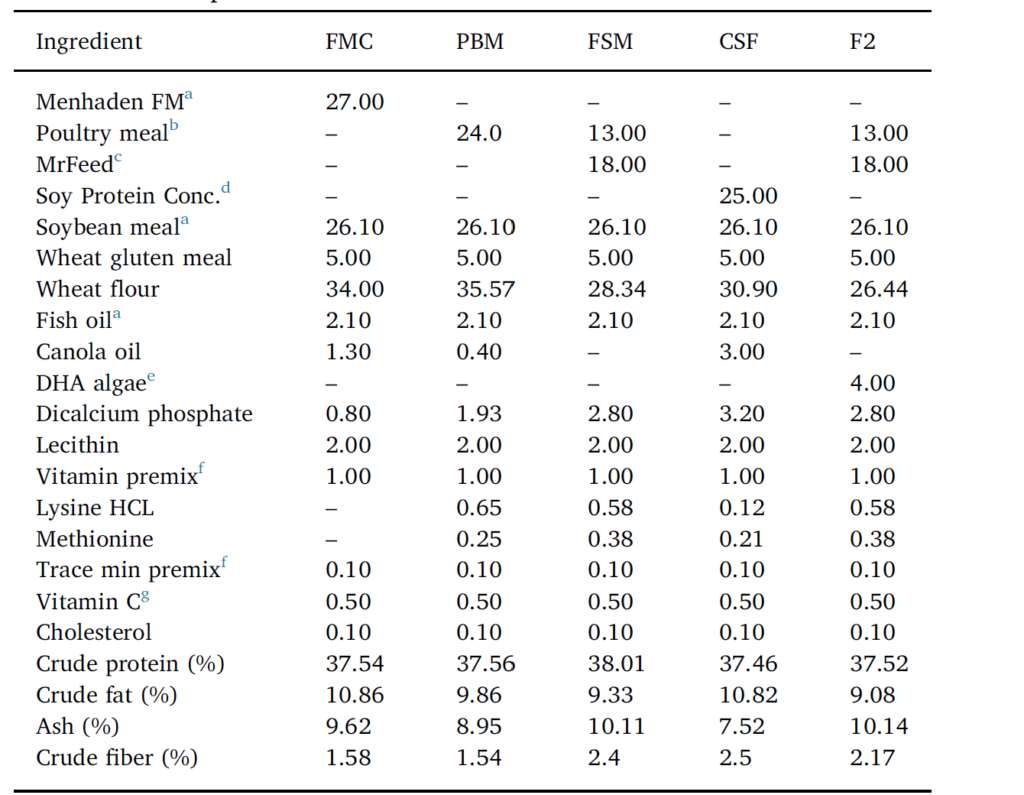
Arkansas, USA) in the poultry by-product feed, and by a blend of PM and fermented SB meal (FS, South Dakota Soy Processors, Volga, SD, USA) in the FSM and FM-free (F2) diet; the latter feed included 4% dry weight DHA algae (Corbion Biotech Inc., Preoria, Illinois, USA). A negative control diet, containing 25% soy protein concentrate (South Dakota Soy Processors, Volga, SD, USA) and 26.1% SBM, represented the CSF feed. 2.1% menhaden fish oil (Daybrook Fisheries, New Orleans, Louisiana, USA) was used as the marine oil in all experimental diets. A commercial feed (Grobest Holdings Ltd., Tao-Yuan City, Taiwan), was also included in the evaluation. Diets were each stored in air-tight plastic containers and refrigerated until use. The feeding trial was undertaken using 24 circular blue plastic tanks of 350 L operational volume. Tanks were part-filled with 250 L 20‰ brackish water and 50 shrimp (78.9 ± 0.9 g total wet wt.) randomly dispersed into each tank. Tanks were then arbitrarily allotted to one of 6 groups (4 tanks per treatment group; 200 shrimp per treatment).
Compositional analyses of feeds were undertaken in accordance with the methods described in AOAC (1994). Amino acid analysis of the diets was undertaken in accordance with the methodology of the European Commission (2009); EC152/ 2009. Free amino acids were extracted with diluted hydrochloric acid. Co-extracted nitrogenous macromolecules were precipitated with sulfosalicylic acid and removed by filtration. The filtered solution was adjusted to pH 2.20. The amino acids were separated by ion exchange chromatography and determined by reaction with ninhydrin with photometric detection at 570 nm.
2.3. Challenge studies
The experimental set-up was based on the methods described in White et al. (2007) and employed 120 L plastic tanks filled with 100 L 20‰ brackish water and serviced by an airlift-based biofilter. The 40- day trial comprised a 1-day adaptation period, a 28-day feeding period, 1-day EMS/AHPND immersion challenge, and a 10-day post-challenge mortality study. The trial was a completely randomized design with treatments being randomly allocated to individual tanks. A stocking density of 20 shrimp per tank was used (200 shrimp m3) and the experiment comprised 8 groups with 4 replicates. The bacterial isolate used was collected from EMS/AHPND infected L. vannamei from a shrimp farm in Loc An, Vung Tau Province, Vietnam by Shrimp VetLab and given the reference code number is LA37 (Lee et al., 2015; Soto- Rodriguez et al., 2015). The isolate was confirmed by PCR (Sirikharin et al., 2015) and bioassay challenge on L. vannamei (Tran et al., 2013). EMS/AHPND challenges were undertaken on both small (3.10 ± 0.05 g) and large (8.34 ± 0.74 g) shrimp. There were no differences in weight between groups in either size range (P > .05).
White et al. (2007) and employed 120 L plastic tanks filled with 100 L 20‰ brackish water and serviced by an airlift-based biofilter. The 40- day trial comprised a 1-day adaptation period, a 28-day feeding period, 1-day EMS/AHPND immersion challenge, and a 10-day post-challenge mortality study. The trial was a completely randomized design with treatments being randomly allocated to individual tanks. A stocking density of 20 shrimp per tank was used (200 shrimp m3) and the experiment comprised 8 groups with 4 replicates. The bacterial isolate used was collected from EMS/AHPND infected L. vannamei from a shrimp farm in Loc An, Vung Tau Province, Vietnam by Shrimp VetLab and given the reference code number is LA37 (Lee et al., 2015; Soto- Rodriguez et al., 2015). The isolate was confirmed by PCR (Sirikharin et al., 2015) and bioassay challenge on L. vannamei (Tran et al., 2013). EMS/AHPND challenges were undertaken on both small (3.10 ± 0.05 g) and large (8.34 ± 0.74 g) shrimp. There were no differences in weight between groups in either size range (P > .05).
2.3.1. Inoculum preparation
Tryptic Soy Broth +2% sodium chloride (TSB+) was inoculated with a consistently virulent strain of V. parahaemolyticus (ShrimpVet reference strain LA37), incubated for 24 h, and added directly into tanks (Tran et al., 2013) to achieve a density of 7.5 × 105 CFU/mL which was expected to kill 90% positive control shrimp (Lethal dose 90%; LD90) within 10-d of challenge as determined by probit analysis (Finney, 1952). Positive control and treatment tanks were subjected to an immersion challenge as previously described (Tran, 2013). Non-inoculated, negative controls were treated with sterile TSB+ added directly to the tanks.
The WSSV challenge differed only in that instead of immersion, animals received per os treatments of 3, rather than immersion for 1 day. The WSSV inoculum used for challenge was collected from infected L. vannamei from Loc An Vung Tau Province, Vietnam by ShrimpVet and given the reference code number 15002. WSSV was confirmed by qPCR detection in multiple samples (Durand and Lightner, 2002). SPF shrimp tissue was used for WSSV negative challenge inoculum. Shrimp, either positive or negative for WSSV, and stored at −80 °C, were thawed and their cuticle removed. In separate procedures for either WSSV positive or negative tissue, tissues were homogenized (50% wt/v) in sterile PBS (Nilsen et al., 2017). WSSV challenges were undertaken on both small (2.66 ± 0.05 g) and large (8.37 ± 0.62 g) shrimp. There were no differences in weight between groups in either size range (P > .05).
2.3.2. Inocula for positive control and treatments
WSSV-infected shrimp tissue was mixed with the control diet (for positive control treatment) and test diets at the ratio of 1:3 (weight/ weight) and stored at -80 °C until use. The inoculum had an average WSSV load of 0.5–1.0 × 109 copies/g and expected LD90, determined by probit analysis for the positive control of 10 days post-challenge. The WSSV inoculum was fed to positive control and treatment groups with 4 meals/day at 5% estimated shrimp body weight for 3 days.
2.3.3. Negative controls
SPF shrimp tissue was mixed with control diet (for negative control) at the ratio of 1:3 (weight/weight) and kept at -80 °C until use. The mixture was fed to negative control as 4 meals/day at 5% estimated shrimp body weight for 3 days of challenge (modified by per os challenge methods described by Han et al., 2017; Di Leonardo et al., 2005; and Wang et al., 1999).
2.3.4. Histological analyses
During challenge trials, two representative moribund shrimp per treatment were collected for histological analyses. Collected histopathology samples were injected with AFA Davidson’s fixative, processed, and stained with hematoxylin and eosin (H&E) using routine histological methods (Bell and Lightner, 1988; Lightner, 1996).
Histological sections were analyzed by light microscopy to examine for damage to the hepatopancreas in EMS/AHPND-exposed and control samples as described previously (Tran et al., 2013; Lightner et al., 2012), and for WSSV lesions (Lightner, 1996; Chou et al., 1995). WSSV lesion severity was assessed accordingly to the G-grading system, with G0 being negative and G4 representing the highest severity of disease (Lightner, 1996). Shrimp in both challenges were fed their respective diet to satiation 4 times daily (5–10% body weight) for the duration of the trial. Feed amount was adjusted based on tank biomass and shrimp feeding behavior. Feed consumption, general behavior and survival throughout the trial was recorded daily at 08.30, 11.00, 14.30 and 20.00 h.
2.4. Calculation and statistical analyses
The parameters used to evaluate the production performance of the shrimp included final weight, weight gain, feed efficiency ratio (FER), protein efficiency ratio (PER) and survival. These were calculated as
follows:
Final weight, g: mean weight of individual shrimp.
Weight gain,%of initial
=([final body weight initial body weight]/[initial body weight]) 100.
FER = (final weight initial weight [g]/dry feed consumed [g]).
PER = ([final weight/initial weight]/protein intake [g]).
Survival = (final population/initial population) × 100.
All data were analyzed using SPSS version 20 (SPSS, Chicago, IL, USA), using one-way analysis of variance (ANOVA) after homogeneity and normality tests. Significant differences among group means were compared using Tukey’s multiple range test. P < .05 was considered statistically significant. Survival curves were assessed using Kaplan- Meier log-rank χ2 tests.
- Results and discussion
Throughout the 8-week feeding trial, water quality values did not differ markedly between tanks and treatments. Moreover, recorded pH, temperature, dissolved oxygen, TAN, nitrite and alkalinity (Table 2), were within previously published ranges supporting growth and survival for L. vannamei (Samocha et al., 2004; Ferreira et al., 2011). No differences (P > .05) were discerned for survival rates across treatment groups, with percent mortalities varying from 27 to 15.5%
Table 2
Average water quality of experimental groups over the duration of a feed trial with Pacific white leg shrimp. No differences between treatments were observed.
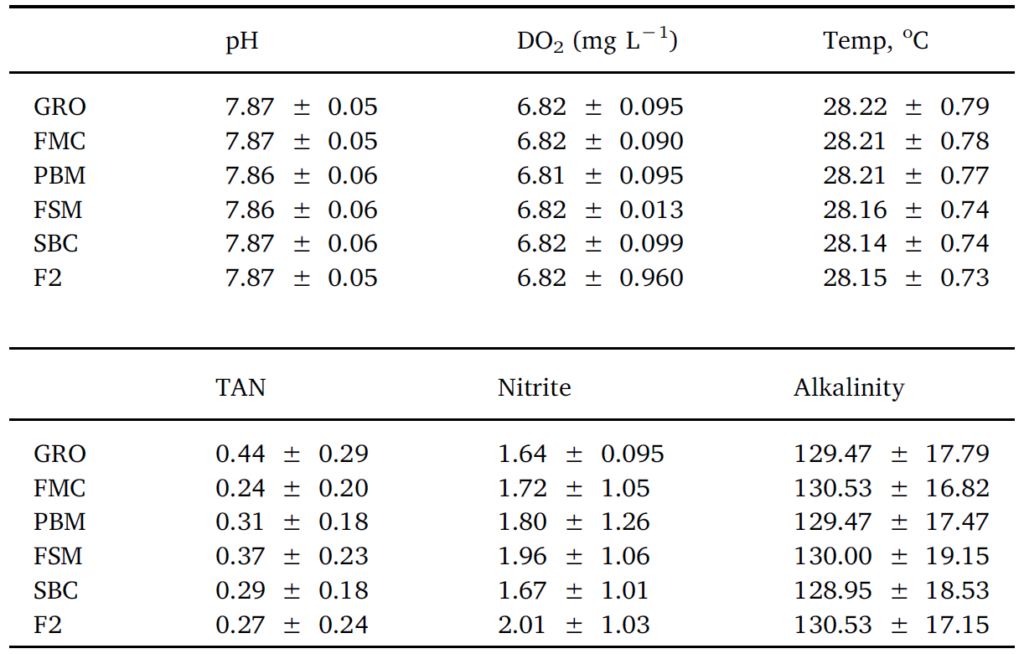
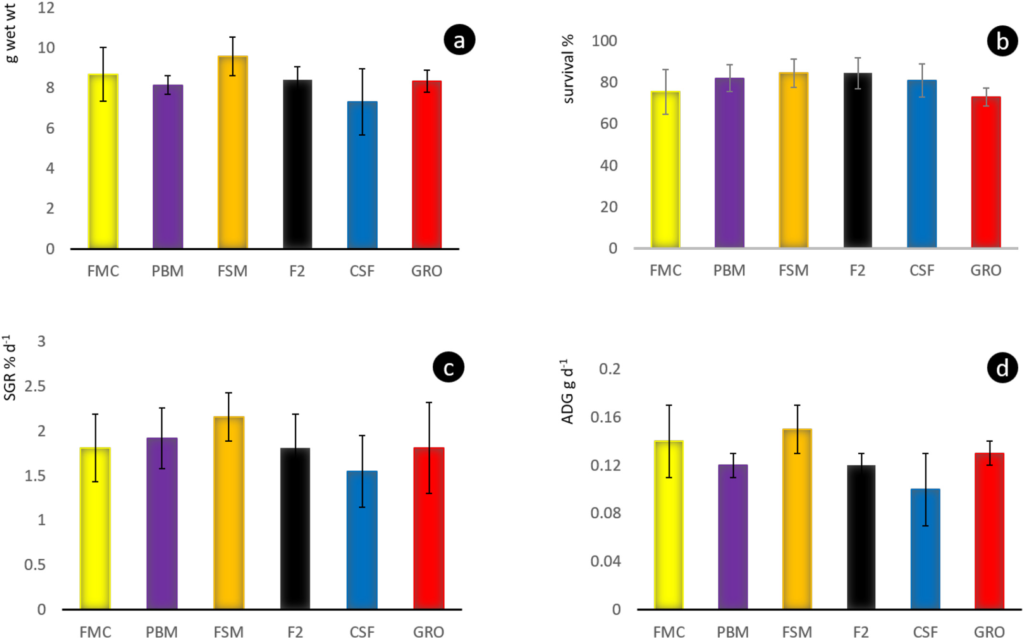
(Fig. 2b). Similar rates of survival of L. vannamei, as recorded herein, have been reported by others where dietary marine protein and oil resources were partly or fully replaced by SBM-based diets and feeds (Lim and Dominy, 1990; Forster et al., 2002; Fox et al., 2011; Samocha et al., 2004; Amaya et al., 2007; Van Nguyen et al., 2018). As well, alternative ingredients, such as single-celled protein (SCP) derived from yeast (McLean et al., 2006), and bacteria (Corynebacterium ammoniagenes; Hamidoghli et al., 2019), and studies with enclosed systems, wherein the water column was biofloc-enhanced (Kuhn et al., 2007, 2008, 2009), report survival of 93% and above. Apart from the FSM we also failed to observe differences (P > .05) between dietary treatments regarding growth performance, including final weights (Fig. 2a), specific growth rate (Fig. 2c), and average daily gain (Fig. 2d). Early studies that substituted marine proteins with terrestrial plant and animal protein in Pacific whiteleg shrimp diets provided variable responses to such exchanges, resulting in differences between trials. However, an increasing number of investigations suggest that these variances may reflect differences in experimental execution, variety of SB employed, quality of technological applications used during downstream processing, and other variables, since 50% and more replacement of FM by SB-based protein appears commonplace (op cit.).
Notwithstanding the possible positive or negative influence of climate change on FM-FO production (Barange et al., 2018), projections suggest that the proportion of fish used for reduction to FM and FO will remain unchanged through 2030 (World Bank, 2013). While overall FM-FO tonnage might be improved marginally, with augmented use of fish processing left-overs (discards, trimmings and offal; Shepherd and Jackson, 2013), and greater utilization of fishery discards (< 6.8 million tonnes per annum; Kelleher, 2005; Zeller et al., 2017; Pérez Roda et al., 2019), the anticipated growth curve for global aquaculture, and especially for shrimp farming, which is projected to double production by 2030 (Kobayashi et al., 2015), will inevitably result in a FM-FO shortfall, leading to a change in real prices of 90% and 70% for FM and FO respectively, by 2030 (Kobayashi et al., 2015). Sustainable growth of the aquaculture sector will depend on the feed and associated industries identifying appropriate alternatives to marine proteins and oils (Sánchez-Muros et al., 2018; Gasco et al., 2018; Glencross et al., 2019). Here, the current study indicates the potential that various processing by-products have as alternative feedstuffs. The trial illustrates little difference in responsiveness of Pacific whiteleg shrimp to the diets over the timeframe of observation used and under conditions of experimentation.
The reaction of whiteleg shrimp, fed in-house and commercial diets for 8+ weeks, to EMS/AHPND immersion challenge, is summarized in Figs. 3 a,b. EMS/AHPND, which was first reported from China, now occurs throughout shrimp-farming areas of the world (op cit.). It can cause 100% mortality of exposed animals within 35-d (Hong et al., 2016) and, in the present experiment, mortality rates of up to 80% were recorded 10-d post-challenge (Fig. 3a). Shrimp groups presented with non-inoculated commercial (control) feed (GRO-) did express fatalities in 2 of the 4 groups also but, in each case, this was a single animal collected at day 2 or just after positive challenges were administered. Poorest rates of survival in inoculated shrimp were observed in F2 fed animals (20.0 ± 22.4%) which differed (P < .05) only to inoculated (GRO+) commercial feed groups. All other challenge groupings returned identical rates of survival (Fig. 3 a,b). The speed at which animals succumbed to challenge was more rapid and ravaging in younger/ smaller shrimp (cf. Fig. 3 a and b; Fig. 5) which experienced over 70% mortality, for all feed types, 4-days post-inoculation. Gross signs and histopathology of EMS/AHPND included a pale-to-white
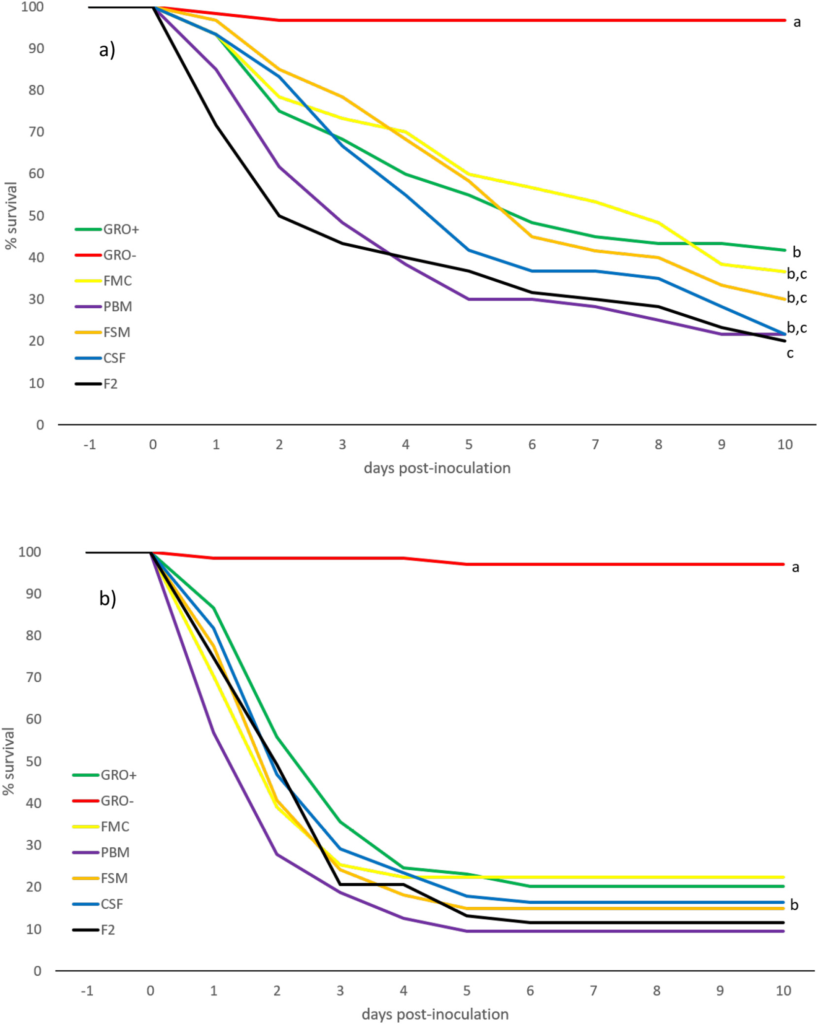
hepatopancreas (HP), significant atrophy of the HP, soft shells, guts with discontinuous, or no contents, and black spots or streaks visible within the HP (due to melanized tubules). In addition, the HP did not squash easily between the thumb and forefinger; likely due to increased presence of fibrous connective tissue and hemocytes (see OIE, 2019). Associated with EMS/AHPND challenge, in both small and large shrimp, was severe HP tubule atrophy and detachment due to tissue degeneration and necrosis (Fig. 6 A, B). Others have reported similar observations, with the suggestion that damage to the stomach is caused by Photorhabdus insect-related binary toxin (PirABVp), which causes epithelial cell detachment and pore formation in the digestive tract, allowing the spread of toxin and bacteria to the HP (Lai et al., 2015). The PirABVp may itself be able to induce signs of morbidity and mortality in infected shrimp.
Over 90 species of crustacea have been reported as carriers of WSSV (Sánchez-Paz, 2010), and its transmission is known to be influenced by a variety of environmental factors, including pH, temperature and salinity. The response of whiteleg shrimp, fed different diets for 8+ weeks, to WSSV delivered per os, over a 3-d period, is summarized in Fig. 4 a,b. Mortality rates of larger shrimp over the 10 d-post-inoculation period varied between a low of 1.7% for negative control groups (non-inoculated commercial feed) to 100% in the positive controls (inoculated commercial feed). Mortalities in the negative control inoculated diet (CSF) were lowest with 76.7% fatalities (Fig. 4a); overall, however, no differences (P > .05) in survival were recorded between inoculated groups. Similar mortality rates for inoculated animals have
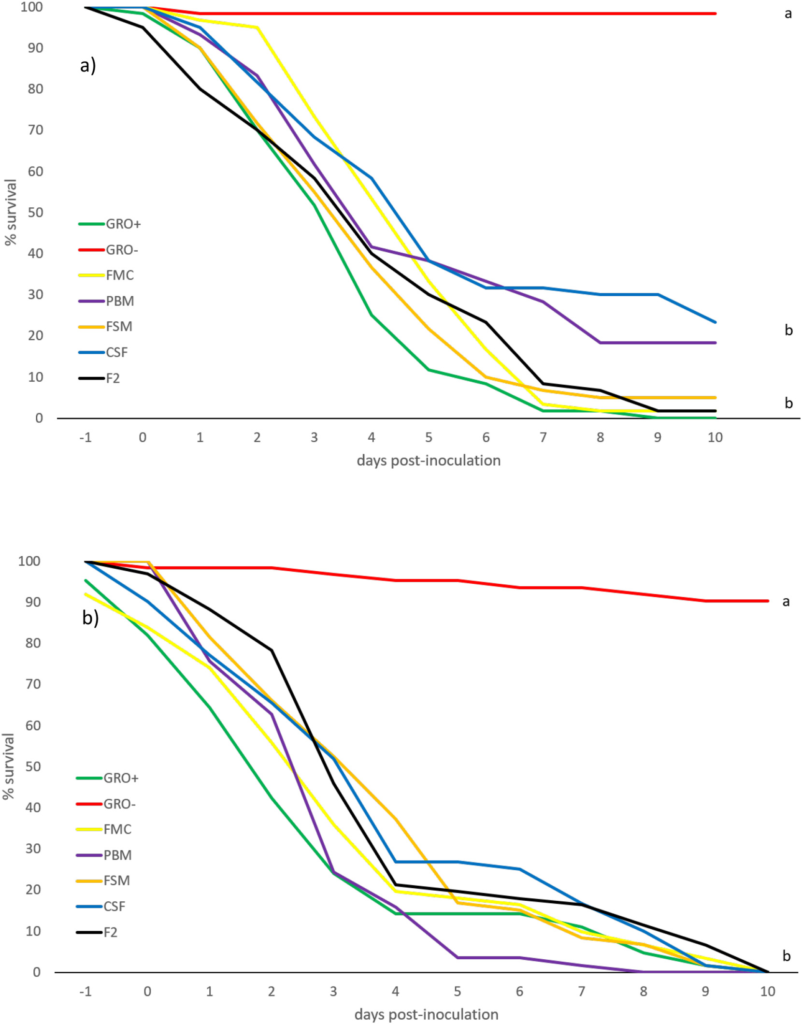
been reported by others (Pérez et al., 2005) although contradictory cases exist, wherein per os inoculation was without effect, even over longer exposure periods (Hasson et al., 2006). The reasons for this disparity are obscure but geographically discrete isolates of WSSV are known to express different levels of irulence, ranging from mild to highly infectious, over variable timeframes of infectivity (Wang et al., 1999). As well, different families of whiteleg shrimp express variance in susceptibility to WSSV (Huang et al., 2011). As with the EMS/AHPND challenge, younger/smaller shrimp were more susceptible to WSSV over the first half of the challenge than larger animals, with the small size range experiencing 100% mortality between 8- and 10-days postinoculation (Fig. 4 b). It is noteworthy that others (e.g., Pérez et al., 2005), have reported higher vulnerability of older animals. These different reports may reflect divergent strains of shrimp employed, variations in pathogenicity of the disease agents, dysbiosis caused by different diets, experimental execution, water quality and otherwise. Gross signs of WSSV revealed that lethargy accompanied cessation of feeding which led to empty guts and stomachs associated with a pale HP. White spots embedded within the exoskeleton represented the most commonly observed clinical sign and, as reported previously (Tran et al., 2013), in small and large shrimp, these spots ranged from barely visible to 3 mm in diameter, sometimes coalescing into larger plates (Fig. 6 C).
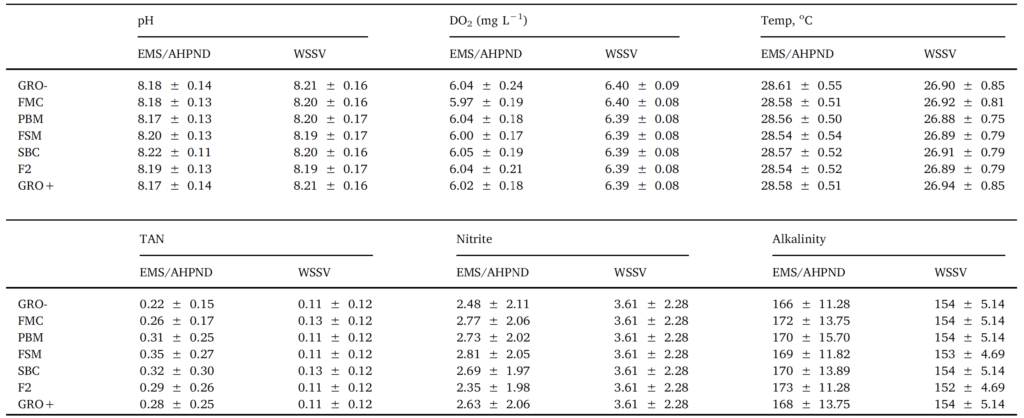
Water quality of experimental groups throughout EMS/AHPND and WSSV challenge studies. No differences in water quality were observed within treatments. The different temperatures employed were optimal based on shrimp susceptibility to EMS/AHPND and WSSV infection.
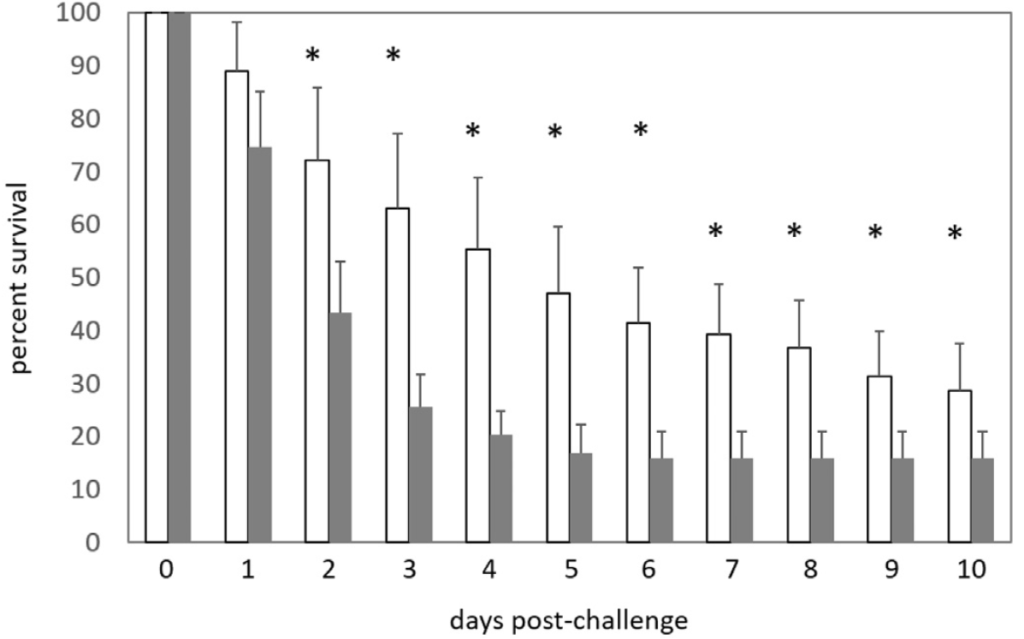
Another prominent pathological sign was reddish-to-pink discoloration of the body. Histological assessment of the stomach and hepatopancreas (Fig. 6 D, E) illustrated classical signs of WSSV infections including the presence of intranuclear inclusion bodies in the stomach region and hepatopancreatic tissue. During both EMS/AHPND and WVVS challenges with large and small shrimp, measured water quality parameters did not differ (P > .05; Table 3).
In this trial, substitution of FM with terrestrial proteins had limited impact on Pacific whiteleg shrimp survival following challenge with two prominent pathogens (Figs. 3 and 4) – no immunologically discernable benefit was derived from investigated diets versus the FM control. In fact, while variable results have been reported, several studies have determined that inclusion of SB-based meals induce high oxidative stress and reduce non-specific immunity in whiteleg shrimp compared to FM-based feeds (Lin and Mui, 2017; Xie et al., 2019). Variability in challenge response may result due to fluctuations in meal composition and quality, the presence of ANFs, feed processing, imbalances in EAA, fatty acids and/or palatability issues. ANFs and imperfect dietary formulation during FM replacement may cause physiological stress in shrimp with negative consequences to the immune system. For example, it is now well-established that an optimal balance of circulating amino acids (AA) is required for the maintenance of homeostasis, and that certain AAs are essential for the regulation of immune function (Wu, 2009). These so-called functional AAs are both essential and non-essential and are involved in the normal operation of phagocytic cells, gene expression, and the production of cytotoxic substances, such as superoxide dismutase and various peroxidases (Li et al., 2007). Imbalances in immunonutrients due to poor dietary formulation, therefore, can cause dysregulation in immune function which may, nonetheless, take some time to become manifest. Indeed, following an 8-week feeding trial in which 25% of dietary FM was replaced by SBM and SB protein concentrate, Xie et al. (2016) reported significantly lower catalase and nitric oxide activity in Pacific whiteleg shrimp hemolymph, suggesting a decreased antioxidant capacity and hence ability to defend against invasive microorganisms. In efforts to reduce such negative effects it has been suggested, numerous times, that dietary constituents used as alternatives to FM should be diverse and selection based upon complimentarity (Davis and Sookying, 2009; see Fig. 7). Alternatively, and/or in addition to FM replacement, strategies for reducing, substituting, or enhancing the nutritive value of alternative proteins include the use of pre-, pro-, and synbiotics (Craig and McLean, 2005; McLean et al., 2006; Chotikachinda et al., 2008; Li et al., 2018; Craig and McLean, 2007), feed attracta nts and stimulants (Nunes et al., 2006), enzymes (McLean et al., 2002), application of novel processing/treatment techniques (Perkins, 1995), and, among others, fatty acid and amino acid supplementations (Chi et al., 2011; Fox et al., 2011; Molina-Poveda et al., 2015; Xie et al., 2017; Moreno-Arias et al., 2018). Regarding the latter, Table 4 summarizes the profile of some essential and non-EAA from test diets. Higher LYS was evident in fermented SB (FSM) feed whereas poultry by-product-based feed (PBM) was lowest in PHE and MET. CSF expressed highest CYS, SER, GLU and PHE and lowest GLY and ALA. Noteworthy was that the commercial diet was highest in dietary concentrations of ALA, VAL, ISO, LEU, HIS and ARG but lowest in MET, GLU and LYS (Table 4). Percentage comparison of some EAA in alternative proteins against fishmeal are presented visually in Fig. 7. When taken together, substitute proteins exceed the levels of all EAA when contrasted against fishmeal, with some proteins exhibiting a 20–40% higher level of specific EAA. Closest EAA profile match was seen in the FSM which corresponded to or exceeded the FMC EAA profile for 6 of 9 EAA and was ≥5% the level of the remainder (Fig. 7; Table 4).
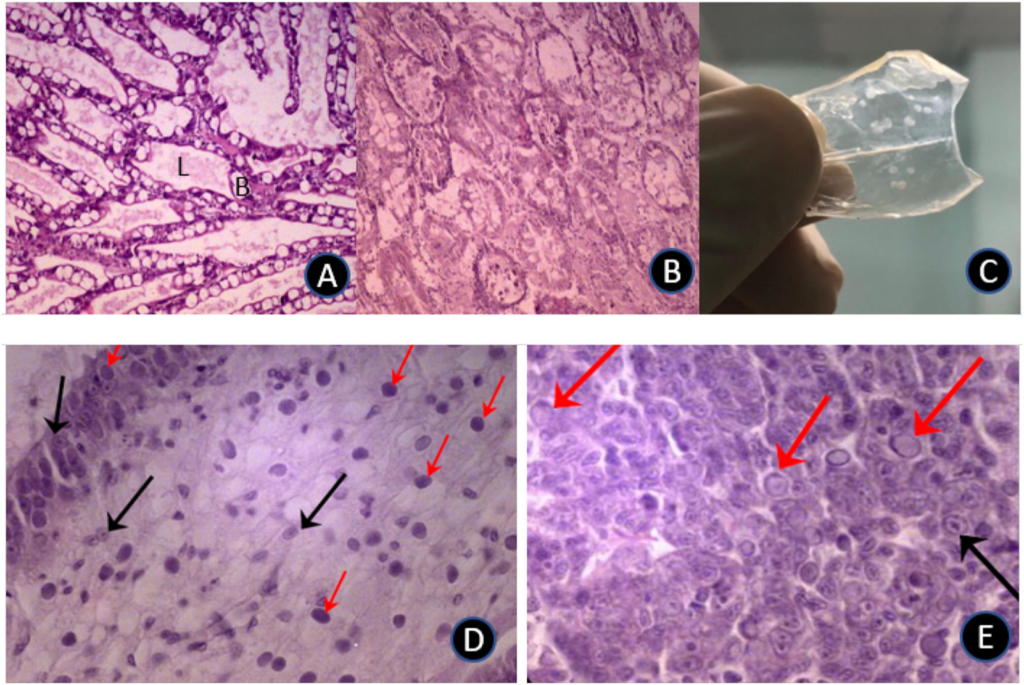
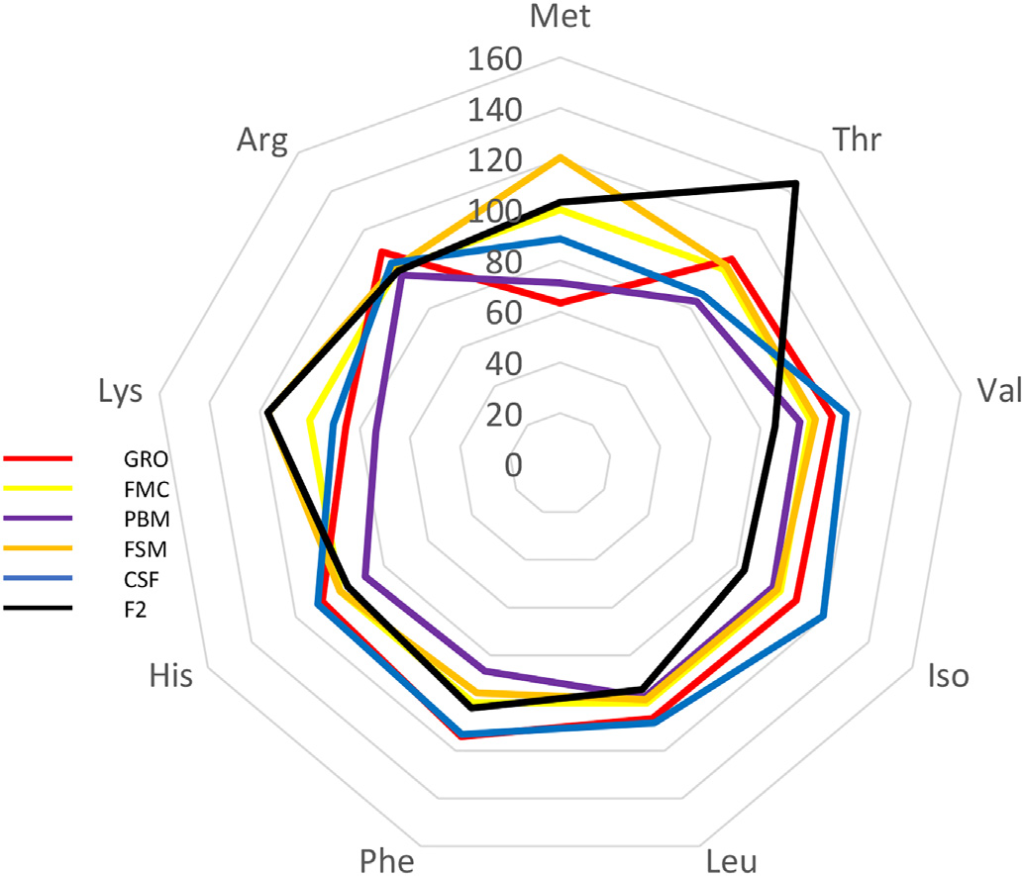
Radar showing relative differences in essential amino acid profiles of varying dietary ingredients used in shrimp feeds. Fishmeal is used as the 100% must point.
It is notable that studies have shown that different processing methods can influence amino acid profiles in SBM (Parsons et al., 1992; Jannathulla et al., 2017), but the dietary profile of amino acids in feeds used here had no measurable impact on shrimp growth (Fig. 3). These experiments, therefore, provide justification for the contention that at least the FM component of L. vannamei diets may be further reduced or even eliminated. However, a precautionary approach should be taken since dietary modification can change the attendant microbial
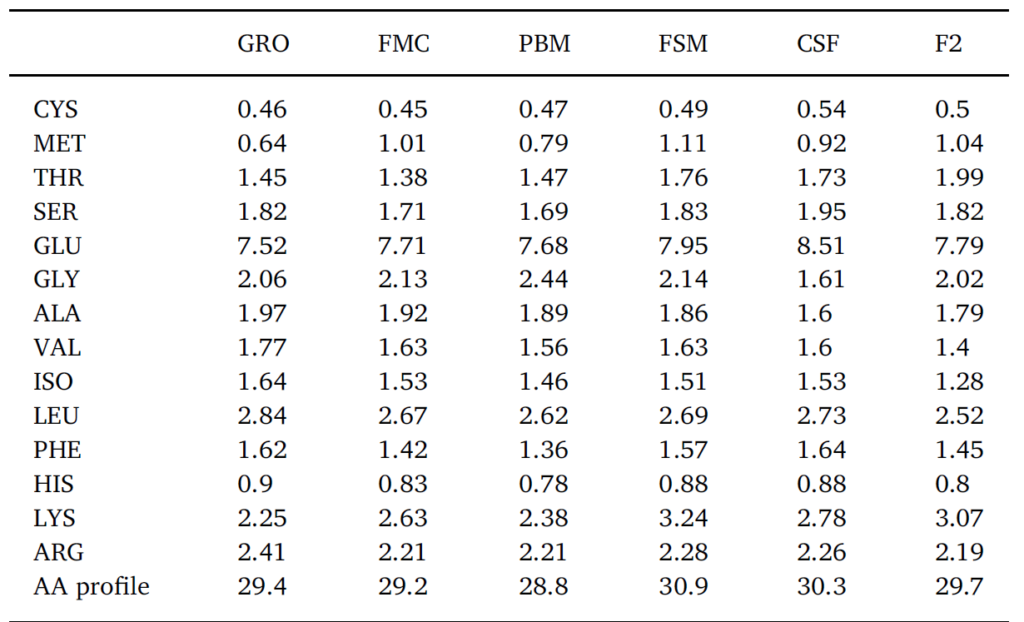
Profile of various measured essential and non-essential amino acids (g/100 g feed) in tested diets.
population of a cultured species, impacting growth and survival through competitive exclusion of pathogens, production of micronutrients and or the secretion of digestive enzymes. Huang et al. (2014), reported adjustments in the bacterial community of the gut of whiteleg shrimp during different stages of development and suggested that the observed variations were likely driven by changes in diet. As identified previously in humans and other animals (Turnbaugh et al., 2009), however, a core gut microbiome, comprising specific bacterial groups (in the case of 2-month old whiteleg shrimp, Proteobacteria, Flavobacteriaceae and Rhodobacteraceae), emerge to predominate all growth stages, irrespective of rearing environment. Cornejo-Granados et al. (2017), further determined that differences in the microbiome of whiteleg shrimp were reflective of previous exposure to disease, but, unlike Huang et al. (2014), they discovered greater microbial diversity in the gut of wild versus healthy farmed shrimp and distinctions in apparent core gut communities between farmed and wild animals. This observation led Cornejo-Granados and colleagues to suggest that whiteleg shrimp domestication might somehow alter gut bacterial loading which could potentially lead to higher risk for disease; corrections to the intestinal microbiome might be made, for example, using probiotic-laced feeds. They offered support for such a contention by pointing out that only wild shrimp from their samples harbored antifungal and antibiotic-producing bacteria and referenced several successful probiotic studies with P. monodon, L. vannamei juveniles and giant freshwater prawn, Macrobrachium rosenbergii (De Man, 1879) larvae. Other work has determined that the gut microbiome of L. vannamei may include strains, corresponding to Pseudoalteromonas and Vibrio genera, able to produce amylases, lipases/esterases and chitinases which might credibly contribute to nutrient digestion (Tzuc et al., 2014). Whether the different diets used in the present trial acted as locomotives of change for the gut microbiome of experimental shrimp remains unknown but nevertheless represents a credible possibility and may partially explain the spread of survival recorded between diets (Figs. 3 and 4). Zhang et al. (2014) report that lipids of varying
fatty acid composition led to potentially beneficial shifts in the intestinal bacterial profile and further studies are needed in this area, incorporating a wider range of feed choices. The suggested use of an array of feedstuffs would not only serve to achieve the nutritional and immune requirements of L. vannamei but also likely influence end product quality and processability. The different feedstuffs used herein each supported reasonable growth (Fig. 2a) and the results add to the growing body of evidence that suggests that whiteleg shrimp may be produced with limited need for marine resource inputs.
Declaration of Competing Interest
The authors have no competing interests.
Acknowledgements
The authors are indebted to the Anthropocene Institute, Palo Alto, CA, USA for funding and support of the current investigations.
References
Alday-Sanz, V., Brock, J., Flegel, T., Mcintosh, R., Bondad-Reantaso, M.G., Salazar, M.,
Subasinghe, R., 2018. Facts, truths and myths about SPF shrimp in aquaculture. Rev.
Aquac. 1–9. https://doi.org/10.1111/raq.12305.
Al-Loman, A., Callow, N.V., Islam, S.M.M., Ju, L.K., 2018. Single-step enzyme processing
of soybeans into intact oil bodies, protein bodies and hydrolyzed carbohydrates.
Process Biochem. 68, 153–164.
Amaya, E., Davis, D.A., Rouse, D.B., 2007. Alternative diets for the Pacific white shrimp
Litopenaeus vannamei. Aquaculture 262, 419–425.
AOAC, 1994. Official Methods of Analysis. Association of Official Analytical Chemists.
Arlington, VA.
Auchterlonie, N., 2018. The continuing importance of fishmeal and fish oil in aquafeeds.
In: Presented at the Aquafarm Conference, Pordenone, Italy, 15–16 February, . www.
iffo.net/iffo-presentations Accessed 19 March 2019.
Barange, M., Bahri, T., Beveridge, M.C.M., Cochrane, K.L., Funge-Smith, S., Poulain, F.
(Eds.), 2018. Impacts of Climate Change on Fisheries and Aquaculture: Synthesis of
Current Knowledge, Adaptation and Mitigation Options. FAO Fisheries and
Aquaculture Technical Paper no 627. FAO, Rome, Italy, pp. 628.
Bell, T.A., Lightner, D.V., 1988. A handbook of normal shrimp histology. In: Special
Publication No. 1, World Aquaculture Society, pp. 114 Baton Rouge, LA.
Cai, J., Zhou, X., Yan, X., Lucente, D., Lagana, C., 2019. Top 10 Species Groups in Global
Aquaculture 2017. FAO, Rome, Italy, pp. 12.
Campbell, K.A., 2010. Protein and Oil Recoveries from Enzyme-Assisted Aqueous
Extraction of Soybeans and Sunflower Seed. Ph.D. Dissertation. Iowa State
University, Ames, Iowa, pp. 173.
Chi, S.Y., Tan, B.P., Lin, H.Z., Mai, K.S., Ai, Q.H., Wang, X.J., Zhang, W.B., Xu, W., Liufu,
Z.G., 2011. Effects of supplementation of crystalline or coated methionine on growth
performance and feed utilization of the Pacific white shrimp, Litopenaeus vannamei.
Aquac. Nutr. 17, e1–e9.
Chotikachinda, R., Lapjatupon, W., Chaisilapasung, S., Sangsue, D., Tantikitti, C., 2008.
Effect of inactive yeast cell wall on growth performance, survival rate and immune
parameters in Pacific white shrimp (Litopenaeus vannamei). Songklanakarin J. Sci.
Technol. 30, 687–692.
Chou, H.Y., Huang, C.Y., Wang, C.H., Chiang, H.C., Lo, C.F., 1995. Pathogenicity of a
baculovirus infection causing white spot syndrome in cultured Penaeid shrimp in
Taiwan. Dis. Aquat. Org. 23, 165–173.
Cornejo-Granados, F., Lopez-Zavala, A.A., Gallardo-Becerra, L., Mendoza-Vargas, A.,
Sánchez, F., Vichido, R., Brieba, L.G., Viana, M.T., Sotelo-Mundo, R.R., Ochoa-Leyva,
A., 2017. Microbiome of Pacific whiteleg shrimp reveals differential bacterial community
composition between wild, aquacultured and AHPND/EMS outbreak conditions.
Sci. Rep. 7, 11783 (2017).
Craig, S.R., McLean, E., 2005. The organic aquaculture movement: a role for NuPro® as an
alternative protein source. In: Lyons, T.P.T., Jaques, K.A. (Eds.), Nutritional
Biotechnology in the Feed and Food Industries. Proceedings of Alltech’s 21st Annual
Symposium, Lexington, Kentucky, USA. Nottingham University Press, UK, pp.
285–293.
Craig, S.R., McLean, E., 2007. Designing organic aquaculture systems: Can we integrate
microbial products and by-products? In: Lyons, T.P.T., Jacques, K. (Eds.), Nutritional
Biotechnology in the Food and Feed Industry. Proceedings of Alltech’s 21st Annual
Symposium Nottingham University Press, UK, Lexington, Kentucky, USA.
Davis, D.A., Sookying, D., 2009. Strategies for reducing and/or replacing fish meal in
production diets for the pacific white shrimp, Litopenaeus vannamei. In: Browdy, C.L.,
Jory, D.E. (Eds.), The Rising Tide, Proceedings of the Special Session on Shrimp
Farming, World Aquaculture 2009. World Aquaculture Society, Baton Rouge
Louisiana, USA, pp. 91–96.
Dawood, M., Koshio, S., Esteban, M., 2017. Beneficial roles of feed additives as immunostimulants
in aquaculture: A review. Rev. Aquac. 10, 950–974.
Di Leonardo, V.A., Bonnichon, V., Roch, P., Parrinello, N., Bonami, J.R., 2005.
Comparative WSSV infection routes in shrimp genera Marsupenaeus and Palaemon. J.
Fish Dis. 28, 565–569.
Durand, S.V., Lightner, D.V., 2002. Quantitative real time PCR for the measurement of
white spot syndrome virus in shrimp. J. Fish Dis. 25, 381–389.
Escobedo-Bonilla, C.M., Alday-Sanz, V., Wille, M., Sorgeloos, P., Pensaert, M.B.,
Nauwynck, H.J., 2008. A review on the morphology, molecular characterization,
morphogenesis and pathogenesis of white spot syndrome virus. J. Fish Dis. 31, 1–18.
European Commission, 2009. Commission regulation (EC) no 152/2009 of 27 January
2009 laying down the methods of sampling and analysis for the official control of
feed. Off. J. Eur. Communities L52, 23–37.
FAO, 2018a. FAO yearbook. In: Fishery and Aquaculture Statistics 2016. FAO, Rome,
Italy, pp. 104.
FAO, 2018b. The State of World Fisheries and Aquaculture: Meeting the Sustainable
Development Goals. FAO, Rome, Italy, pp. 210.
Ferreira, N.C., Bonneti, C., Seiffert, W.Q., 2011. Hydrological and water quality indices as
management tools in marine shrimp culture. Aquaculture 318, 425–433.
Finney, D.J., 1952. Probit analysis (2nd Ed). J. Inst. Actua. 78, 388–390.
3 al 6 de Septiembre delForster, I.P., Dominy, W., Tacon, A.J.G., 2002. The use of concentrates
and other soy products in shrimp feeds. In: Cruz-Suárez, L.E., Ricque-Marie,
D., Tapia-Salazar, M., Gaxiola-Cortés, M.G., Simoes, N. (Eds.), Avances en Nutrición
Acuícola VI. Memorias del VI Simposium Internacional de Nutrición Acuícola.
Cancún, Quintana Roo, México.
Fox, J.M., Humes, M., Davis, D.A., Lawrence, A.L., 2011. Evaluation of methionine supplements
and their use in grain-based feeds for Litopenaeus vannamei. J. Wprld Aquac.
Soc. 42, 676–686.
Gasco, L., Gai, F., Maricchiolo, G., Genovese, L., Ragonese, S., Bottari, T., Caruso, G.,
- Fishmeal alternative protein sources for aquaculture feeds. In: Feeds for the
Aquaculture Sector. Current Situation And Alternative Sources. Springer Briefs in
Molecular Science. Springer, Cham, Switzerland, pp. 1–28.
Gatlin, D.M., Barrows, F.T., Brown, P., Dabrowski, K., Gaylord, T.G., Hardy, R.W.,
Herman, E., Hu, G., Krogdahl, Å., Nelson, R., Overturf, K., Rust, M., Sealey, W.,
Skonberg, D., Souza, E.J., Stone, D., Wilson, R., Wurtele, E., 2007. Expanding the
utilization of sustainable plant products in aquafeeds: a review. Aquac. Res. 38,
551–579.
Glencross, B.D., Baily, J., Berntssen, M.H.G., Hardy, R., MacKenzie, S., Tocher, D.R., - Risk assessment of the use of alternative animal and plant raw material resources
in aquaculture feeds. Rev. Aquac. 11, 1–56.
Hamidoghli, A., Yun, H., Won, S., Kim, S., Farris, N.W., Bai, S.C., 2019. Evaluation of a
single-cell protein as a dietary fish meal substitute for whiteleg shrimp Litopenaeus
vannamei. Fish. Sci. 85, 147–155.
Han, Y., Li, F., Xu, L., Yang, F., 2017. A VP24-truncated isolate of white spot syndrome
virus is inefficient in per os infection. Vet. Res. 48, 87.
Hasson, K.W., Fan, Y., Reisinger, T., Venuti, J., Varner, P.W., 2006. White-spot syndrome
virus (WSSV) introduction into the Gulf of Mexico and Texas freshwater systems
through imported, frozen bait-shrimp. Dis. Aquat. Org. 71, 91–100.
Hong, X., Lu, L., Xu, D., 2016. Progress in research on acute hepatopancreatic necrosis
disease (AHPND). Aquac. Int. 24, 577–593.
Hossain, M.S., Koshio, S., Kestemont, P., 2019. Recent advances of nucleotide nutrition
research in aquaculture: a review. Rev. Aquac. https://doi.org/10.1111/raq.12370.
Huang, Y.-C., Yin, Z.-X., Ai, H.-S., Huang, X.-D., Li, S.-D., Weng, S.-P., He, J.-G., 2011.
Characterization of WSSV resistance in selected families of Litopenaeus vannamei.
Aquaculture 311, 54–60.
Huang, Z., Li, X., Wang, L., Shao, Z., 2014. Changes in the intestinal bacterial community
during the growth of white shrimp, Litopenaeus vannamei. Aquac. Res. 47,
1737–1746.
Hulefeld, R., Habte-Tsion, H.-M., Lalgudi, R.S., McGraw, B., Cain, R., Allen, K.,
Thompson, K.R., Tidwell, J.H., Kumar, V., 2018. Nutritional evaluation of an improved
soybean meal as a fishmeal replacer in the diet of Pacific white shrimp,
Litopenaeus vannamei. Aquac. Res. 49, 1414–1422.
Jamal, M.T., Abdulrahman, I.A., Al Harbi, M., Chithambaran, S., 2019. Probiotics as alternative
control measures in shrimp aquaculture: a review. J Appl Biol Biotechnol 7, - 69–77.
- Jannathulla, R., Syama Dayal, J., Vasanthakumar, D., Ambasankar, K., Muralidhar, M.,
- Effect of fermentation methods on amino acids, fiber fractions and anti-nutritional
factors in different plant protein sources and essential amino acid index for
Penaeus vannamei Boone, 1931. Indian J Fish 64, 40–47.
Karunasagar, I., Karunasagar, I., 2018. Ecology, virulence factors and global spread of
Vibrio parahaemolyticus. Asian Fish Sci 31S, 15–28.
Kelleher, K., 2005. Discards in the World’s Marine Fisheries: An update. FAO Fish Tech
Pap No. 470. FAO, Rome, Italy, pp. 131.
Kobayashi, M., Msangi, S., Batka, M., Vannuccini, S., Dey, M.M., Anderson, J.L., 2015.
Fish to 2030: the role and opportunity for aquaculture. Aquac. Econ. Manag. 19,
282–300.
Kuhn, D.D., Boardman, G.D., Craig, S.R., Flick, G.J., Mclean, E., 2007. Evaluation of tilapia
effluent with ion supplementation for marine shrimp production in a recirculating
aquaculture system. J. World Aquacult. Soc. 38, 74–84.
Kuhn, D.D., Boardman, G.D., Craig, S.R., Flick Jr., G.J., McLean, E., 2008. Use of microbial
flocs generated from tilapia effluent as a nutritional supplement for
Litopenaeus vannamei in recirculating aquaculture systems. J. World Aquacult. Soc.
39, 72–82.
Kuhn, D.D., Boardman, G.D., Lawrence, A.L., Marsh, L., Flick Jr., G.J., 2009. Microbial
floc meal as a replacement ingredient for fish meal and soybean protein in shrimp
feed. Aquaculture 296, 51–57.
Kumar, G., Engle, C.R., 2016. Technological advances that led to growth of shrimp,
salmon, and tilapia farming. Rev. Fish. Sci. Aqua. 24, 136–152.
Lagos, L.V., Stein, H.H., 2017. Chemical composition and amino acid digestibility of
soybean meal produced in the United States, China, Argentina, Brazil, or India. J.
Anim. Sci. 95, 1626–1636.
Lai, H.-C., Ng, T.H., Ando, M., Lee, C.-T., Chen, I.-T., Chuang, J.-C., Mavichak, R., et al., - Pathogenesis of acute hepatopancreatic necrosis disease (AHPND) in shrimp.
Fish Shellf Immunol 47, 1006–1014.
Lee, C.-T., Chen, I.-T., Yang, Y.-T., Ko, T.-Z., Huang, Y.-T., Huang, J.-Y., et al., 2015. The
opportunistic marine pathogen Vibrio parahaemolyticus becomes virulent by acquiring
a plasmid that expresses a deadly toxin. Proc. Natl. Acad. Sci. U. S. A. 112,
10798–10803.
Li, P., Yin, Y.-L., Li, D., Kim, S.W., Wu, G., 2007. Amino acids and immune function. Br. J.
Nutr. 98, 237–252.
Li, E., Xu, C., Wang, X., Wang, S., Zhao, Q., Zhang, M., Qin, J.G., Chen, L., 2018. Gut
microbiota and its modulation for healthy farming of Pacific white shrimp Litopenaeus
vannamei. Rev Fish Sci Aquacu. https://doi.org/10.1080/23308249.2018.1440530.
Li, C., Wengh, S., He, J., 2019. WSSV-host interaction: host response and immune evasion.
Fish Shellf Immunol 84, 558–571.
Lightner, D.V., 1996. A Handbook of Shrimp Pathology and Diagnostic Procedures for
Diseases of Cultured Penaeid Shrimp. World Aquaculture Society, Baton Rouge,
Louisiana, USA, pp. 300.
Lightner, D.V., Redman, R.M., Pantoja, C.R., Noble, B.I., Tran, Loc, 2012. Early mortality
syndrome affects shrimp in Asia. Global Aquacult Adv January/February 2012, 40.
Lim, C., Dominy, W., 1990. Evaluation of soybean meal as a replacement for marine
animal protein in diets for shrimp (Penaeus vannamei). Aquaculture 87, 53–63.
Lin, Y.-H., Mui, J.-J., 2017. Comparison of dietary inclusion of commercial and fermented
soybean meal on oxidative status and non-specific immune responses in white
shrimp, Litopenaeus vannamei. Fish Shellf Immunol 63, 208–212.
Luis-Villasenor, I.E., Castellanos-Cervantes, T., Gomez-Gil, B., Carrillo-Garcia, A.E.,
CampaCordova, A.I., Ascencio, F., 2013. Probiotics in the intestinal tract of juvenile
whiteleg shrimp Litopenaeus vannamei: modulation of the bacterial community. World
J. Microbiol. Biotechnol. 29, 257–265.
Malcorps, M., Kok, B., van’t Land, M., Fritz, M., van Doren, D., Servin, K., van der Heijden,
P., Palmer, R., Auchterlonie, N.A., Rietkerk, M., Santos, M.J., Davies, S., 2019. The
sustainability conundrum of fishmeal substitution by plant ingredients in shrimp
feeds. Sustainability 11, 1212.
McLean, E., Craig, S.R., Goddard, S.J., Al-Oufi, H.S., Wille, K., 2002. Exoenzymes in
aquafeeds with particular reference to microbial phytase: a review. Ribarstvo 60,
15–28.
McLean, E., Reid, B., Fegan, D., Kuhn, D., Craig, S.R., 2006. Total replacement of fishmeal
with an organically certified yeast-based protein in Pacific white shrimp (Litopenaeus
vannamei) diets: laboratory and field trials. Ribarstvo 64, 47–58.
Molina-Poveda, C., Cárdenas, R., Jover, m., 2015. Evaluation of amaranth (Amaranthus
caudatus L.) and quinoa (Chenopodium quinoa) protein sources as partial substitutes
for fish meal in Litopenaeus vannamei grow-out diets. Aquac. Res. 48, 822–835.
Morales-Covarrubias, M.S., Cuéllar-Anjel, J., Varela-Mejías, A., Elizondo-Ovares, C., - Shrimp bacterial infections in Latin America: a review. Asian Fish Sci 31S,
76–87.
Moreno-Arias, A., López-Elías, J.A., Martínez-Córdova, L.R., Ramírez-Suárez, J.C.,
Carvallo-Ruiz, M.G., García-Sánchez, G., Lugo-Sánchez, M.E., Miranda-Baeza, A., - Effect of fishmeal replacement with a vegetable protein mixture on the amino
acid and fatty acid profiles of diets, biofloc and shrimp cultured in BFT system.
Aquaculture 483, 53–62.
Moss, S.M., Moss, D.R., Arce, S.M., Lightner, D.V., Lotz, J.M., 2012. The role of selective
breeding and biosecurity in the prevention of disease in penaeid shrimp aquaculture.
J. Invertebr. Pathol. 110, 247–250.
Mukherjee, R., Chakraborty, R., Dutta, A., 2016. Role of fermentation in improving nutritional
quality of soybean meal – A review. Asia-Aust J Anim Sci 29, 1523–1529.
Muthukrishnan, S., Defoirdt, T., Ina-Salwany, M.Y., Yusoff, F.M., Shariff, M., Ismail, S.I.,
Natrah, I., 2019. Vibrio parahaemolyticus and Vibrio harveyi causing acute
Hepatopancreatic necrosis disease (AHPND) in Penaeus vannamei (Boone,1931) isolated
from Malaysian shrimp ponds. Aquaculture 151, 234227.
Naylor, R.L., Hardy, R.W., Bureau, D.P., Chiu, A., Elliott, M., Farrell, A.P., Forster, I.,
Gatlin, D.M., Goldburg, R.J., Hua, K., Nichols, P.D., 2009. Feeding aquaculture in an
era of finite resources. Proc. Natl. Acad. Sci. U. S. A. 106, 15103–15110.
Nilsen, P., Karlsen, M., Sritunyalucksana, K., Thitamadee, S., 2017. White spot syndrome
virus VP28 specific double-stranded RNA provides protection through a highly focused
siRNA population. Sci. Rep. 7, 1028. https://doi.org/10.1038/s41598-017-
01181-w.
Nunes, A., Sá, M.V.C., Andriola-Neto, F.F., Lemos, D., 2006. Behavioral response to selected
feed attractants and stimulants in Pacific white shrimp, Litopenaeus vannamei.
Aquaculture 260, 244–254.
Oakey, J., Smith, C., Underwood, D., Afsharnasab, M., Alday-Sanz, V., Dhar, A.,
Sivakumar, S., Sahul, H., Beattie, K., Crook, A., 2019. Global distribution of white
spot syndrome virus genotypes determined using a novel genotyping assay. Arch.
Virol. 164, 2061–2082.
OIE, 2019. Manual of diagnostic tests for aquatic animals (2019). https://www.oie.int/
standard-setting/aquatic-manual/access-online/ accessed 2/19.
Parsons, C.M., Hashimoto, K., Wedekind, K.J., Han, Y., Baker, D.H., 1992. Effect of
overprocessing on availability of amino acids and energy in soybean meal. Poult. Sci.
71, 133–140.
A third assessment of global marine fisheries discards. In: Pérez Roda, M.A., Gilman, E.,
Huntington, T., Kennelly, S.J., Suuronen, P., Chaloupka, M., Medley, P. (Eds.), FAO
Fish Aqua Tech Pap T633. FAO, Rome Italy, pp. 79.
Pérez, F.C., Volckaert, F., Calderón, J.A., 2005. Pathogenicity of white spot syndrome
virus on postlarvae and juveniles of Penaeus (Litopenaeus) vannamei. Aquaculture 250,
586–591.
Perkins, E.G., 1995. Composition of soybeans and soybean products. In: Erickson, D.R.
(Ed.), Practical Handbook of Soybean Processing and Utilization. AOCS-Elsevier
Press, pp. 9–28 130.
Salazar, M., Aragon, L., Gúiza, L., Caraballo, X., Granja, C., 2010. Emerging diseases in
shrimp culture: overview of viral and bacterial diseases in the Americas. Asian Fish
Sci 23, 427–433.
Salin, K.R., Arun, V.V., Nair, C.M., Tidwell, J.H., 2018. Sustainable aquafeed. In: Hai, F.I.,
Visvanathan, C., Boopathy, R. (Eds.), Sustainable aquaculture. Springer, Cham,
Switzerland, pp. 123–151 327 pp.
Samocha, T.M., Davis, D.A., Saoud, I.P., DeBault, K., 2004. Substitution of fish meal by
co-extruded soybean poultry by-product meal in practical diets for the Pacific white
shrimp, Litopenaeus vannamei. Aquaculture 231, 197–203.
Sánchez-Muros, M.J., Renteria, P., Vizcaino, A., Barroso, F.G., 2018. Innovative protein
sources in shrimp (Litopenaeus vannamei) feeding. Rev. Aquac. https://doi.org/10.
1111/raq.12312.
Sánchez-Paz, A., 2010. White spot syndrome virus: an overview on an emergent concern.
Vet. Res. 41, 43.
Shepherd, C.J., Jackson, A.J., 2013. Global fishmeal and fish-oil supply: inputs, outputs
and markets. J. Fish Biol. 83, 1046–1066.
Shinn, A.P., Pratoomyot, J., Griffiths, D., Trong, T., Jiravanichpaisal, P., Briggs, M., 2018.
Asian shrimp production and the economic costs of disease. Asian Fish Sci 31S,
29–58.
Sirikharin, R., Taengchaiyaphum, S., Sanguanrut, P., Chi, T.D., Mavichak, R.,
Proespraiwong, P., Nuangsaeng, B., Thitamadee, S., Flegel, T.W., Sritunyalucksana,
K., 2015. Characterization and PCR detection of binary, Pir-like toxins from Vibrio
parahaemolyticus isolates that cause acute hepatopancreatic necrosis disease
(AHPND) in shrimp. PLoS One 10, e0126987. https://doi.org/10.1371/journal.pone.
0126987.
Sitjà-Bobadilla, A., Peña-Llopis, S., Gómez-Requeni, P., Médale, F., Kaushik, S., Pérez-
Sánchez, J., 2005. Effect of fish meal replacement by plant protein sources on nonspecific
defence mechanisms and oxidative stress in gilthead sea bream (Sparus
aurata). Aquaculture 249, 387–400.
Soto-Rodriguez, S.A., Gomez-Gil, B., Lozano-Olvera, R., Betancourt-Lozano, M., Morales-
Covarrubias, M.S., 2015. Field and experimental evidence of Vibrio parahaemolyticus
as the causative agent of acute hepatopancreatic necrosis disease of cultured shrimp
(Litopenaeus vannamei) in northwestern Mexico. Appl. Environ. Microbiol. 81,
1689–1699.
Tacon, A.G.J., Metian, M., 2015. Feed matters: satisfying the feed demand of aquaculture.
Rev Fish Sci Aquac 23, 1–10.
Tang, K.F., Pantoja, C.R., Redman, R.M., Han, J.E., Tran, L.H., Lightner, D.V., 2015.
Development of in situ hybridization and PCR assays for the detection of
Enterocytozoon hepatopenaei (EHP), a microsporidian parasite infecting penaeid
shrimp. J. Invertebr. Pathol. 130. https://doi.org/10.1016/j.jip.2015.06.009.
Thitamadee, S., Prachumwat, A., Srisala, J., Jaroenlak, P., Salachan, P.V.,
Sritunyalucksana, K., Flegel, T.W., Itsathitphaisarn, O., 2016. Review of current
disease threats for cultivated penaeid shrimp in Asia. Aquaculture 452, 69–87.
Toledo, A., Frizzo, L., Signorini, M., Bossiere, P., Arenala, A., 2019. Impact of probiotics
on growth performance and shrimp survival: a meta-analysis. Aquaculture 500,
196–205.
Tran, H.L., 2013. Determination, characterization, and control measures of the agent
causing early mortality syndrome (EMS) also known as acute hepatopancreatic necrosis
syndrome (AHPNS) in farmed penaeid shrimp. PhD. Thesis. The University of
Arizona, USA.
Tran, L., Nunan, L., Redman, R.M., Mohney, L.L., Pantoja, C.R., Fitzsimmons, K., Lightner,
D.V., 2013. Determination of the infectious nature of the agent of acute hepatopancreatic
necrosis syndrome affecting penaeid shrimp. Dis. Aquat. Org. 105, 45–55.
Turnbaugh, P.J., Hamady, M., Yatsunenko, T., Cantarel, B.L., Duncan, A., Ley, R.E., Sogin,
M.L., Jones, W.J., Roe, B.A., Affourtit, J.P., 2009. A core gut microbiome in obese and
lean twins. Nature 457, 480–484.
Tzuc, J.T., Escalante, D.R., Herrera, R.R., Cortés, G.G., Ortiz, M.L.A., 2014. Microbiota
from Litopenaeus vannamei: digestive tract microbial community of Pacific white
shrimp (Litopenaeus vannamei). 2014 SpringerPlushttps://doi.org/10.1186/2193- - 1801-3-280. 3, 280.
- Van Nguyen, N., Hoang, L., Van Khanh, T., Duy Hai, P., Hung, L.T., 2018. Utilization of
- fermented soybean meal for fishmeal substitution in diets of Pacific white shrimp
- (Litopenaeus vannamei). Aquac. Nutr. 24, 1092–1100.
- Wang, Q.L., White, B.M., Redman, R., Lightner, D.V., 1999. Per os challenge of Litopenaeus
- vannamei postlarvae and Farfantepenaeus duorarum juveniles with six geographic
- isolates of white spot syndrome virus. Aquaculture 170, 179–194.
- White, B.L., Schofield, P.J., Poulos, B.T., Lightner, D.V., 2007. A laboratory challenge
- method for estimating Taura syndrome virus resistance in selected lines of Pacific
- white shrimp Litopenaeus vannamei. J. World Aquacult. Soc. 33, 341–348.
- World Bank, 2013. Fish to 2030. Prospects for fisheries and aquaculture. In: World Bank
- Report Number 83177-GLB. Agriculture and Environmental Services Discussion
- Paper 03. World Bank, Washington DC, USA, pp. 80.
- Wu, G., 2009. Amino acids: metabolism, functions, and nutrition. Amino Acids 37, 1–17.
- Xie, S., Liu, Y.-J., Zeng, S., Niu, J., Tian, L.-X., 2016. Partial replacement of fish-meal by
- soy protein concentrate and soybean meal based protein blend for juvenile Pacific
- white shrimp, Litopenaeus vannamei. Aquaculture 464, 296–302.
- Xie, J.-J., Lemme, A., He, J.-Y., Yin, P., Figueiredo-Silva, C., Liu, Y.-J., Xie, S.-W., Niu, J.,
- Tian, L.-X., 2017. Fishmeal levels can be successfully reduced in white shrimp
- (Litopenaeus vannamei) if supplemented with DL-methionine (DL-met) or DLMethionyl-
- DL-methionine (met-met). Aquac. Nutr. 24, 1144–1152.
- Xie, S., Wei, D., Yin, P., Zheng, L., Guo, T., Liu, Y., Tian, L., Niu, J., 2019. Dietary replacement
- of fish-meal impaired protein synthesis and immune response of juvenile
- Pacific white shrimp, Litopenaeus vannamei at low salinity. Comp. Biochem. Physiol.
- B: Biochem. Mol. Biol. 228, 26–33.
- Zeller, D., Cashion, T., Palomares, M., Pauly, D., 2017. Global marine fisheries discards: A
- synthesis of reconstructed data. Fish Fish. 19, 30–39.
- Zhang, Y., Wu, Y., Jiang, D., Qin, J., Wang, Y., 2014. Gamma-irradiated soybean meal
- replaced more fish meal in the diets of Japanese seabass (Lateolabrax japonicus).
- Anim. Feed Sci. Technol. 197, 155–163.